|
NEW SCIENCES FOR A NEW ERA:
Mathematical, Physical and Chemical Discoveries of
Ruggero Maria Santilli
I. Gandzha and J Kadeisvili
PRINTED VERSION
I. Gandzha and J Kadeisvili, New Sciences for a New Era:
Mathematical, Physical and Chemical Discoveries of
Ruggero Maria Santilli,
Sankata Printing Press, University of Kathmandy, Nepal (2011),
Free pdf download
http://www.santilli-foundation.org/docs/RMS.pdf
CHAPTER 4:
DISCOVERIES IN
CHEMISTRY AND BIOLOGY
4.1. INTRODUCTION
4.1A. Lack of exact character of quantum mechanics for the hydrogen
molecule (1978)
4.1B. Insufficiencies of the quantum chemical notion of valence
(1978)
4.1C. Insufficiencies of screened Coulomb potentials (1978))
4.1D. Classification of hadronic chemistry (2000)
4.1E. Basic literature
4.2. HADRONIC CHEMISTRY
4.2A. Animalu-Santilli Cooper pair (1995)
4.2B. Santilli-Shillady strong valence bond (1999)
4.2C.The isoelectronium (1999)
4.2D. The hydrogen molecule (1999)
4.2E. The water molecule (2000)
4.3. THE NEW CHEMICAL SPECIES OF SANTILLI MAGNECULES
4.3A. Historical notes
4.3B. Conception of Santilli magnecules (1998)
4.3C. Detection of Santilli magnecules (1998)
4.3D. Magnecular structure of H3 and O3
(1998)
4.3E. Magnecular structure of liquids and solids (1998)
4.4 INDUSTRIAL REALIZATION OF FUELS WITH MAGNECULAR STRUCTURE
4.4A. Catastrophic forecasts facing mankind
4.4B. Santilli hadronic reactors (1998)
4.4C. Industrial realization of MagneGas fuel (1998)
4.4D. Industrial realization of the HHO fuel (2006)
4.4E. Industrial realization of MagneHydrogen fuel (2003)
4.4F. Molecular and magnecular combustions (1998).
4.5. SANTILLI DISCOVERY IN BIOLOGY
4.5A. Historical notes
4.5B. Deformability, irreversibility, and multi-valuedness of
biological structures.
4.5C. Representation of biological structures via Santilli's
deformable, irreversible and multi-valued hypermathematics
4.5D. Hypermolecules, hypermagnecules and hyperliquids
4.5E. Deciphering the DNA code?
4.5F. Understanding the DNA structure?
4.5G. A future new cure for cancer?
4.5H. Cloonan's advances in Santilli Magnecules
CHAPTER 4:
SANTILLI DISCOVERIES IN
CHEMISTRY AND BIOLOGY
4.1. INTRODUCTION
4.1A. Lack of exact character of quantum mechanics for the hydrogen
molecule (1978)
As recalled in Section 1.9, quantum chemistry has indeed permitted the
achievement during the 20th century of historical advances in material
and equipment of our daily lives. Nevertheless, it is the fate of all
theories to admit broader formulations rendered necessary by
insufficiencies of pre-existing theories or by the advent of basically
new conditions for which preceding theories were not intended for.
As set in history, quantum mechanics provided a representation of the
structure of one hydrogen atom with an incredible accuracy,
essentially to the desired decimal value. However, when studying
two hydrogen atoms bonded in the hydrogen molecule H2
= H-H (where the dash "-" represents valence bond hereon), the preceding
accuracy is lost due to the historical inability to represent a residual
2 % of the binding energy from unadulterated quantum axioms, with much
greater inaccuracies when passing to more complex molecules.
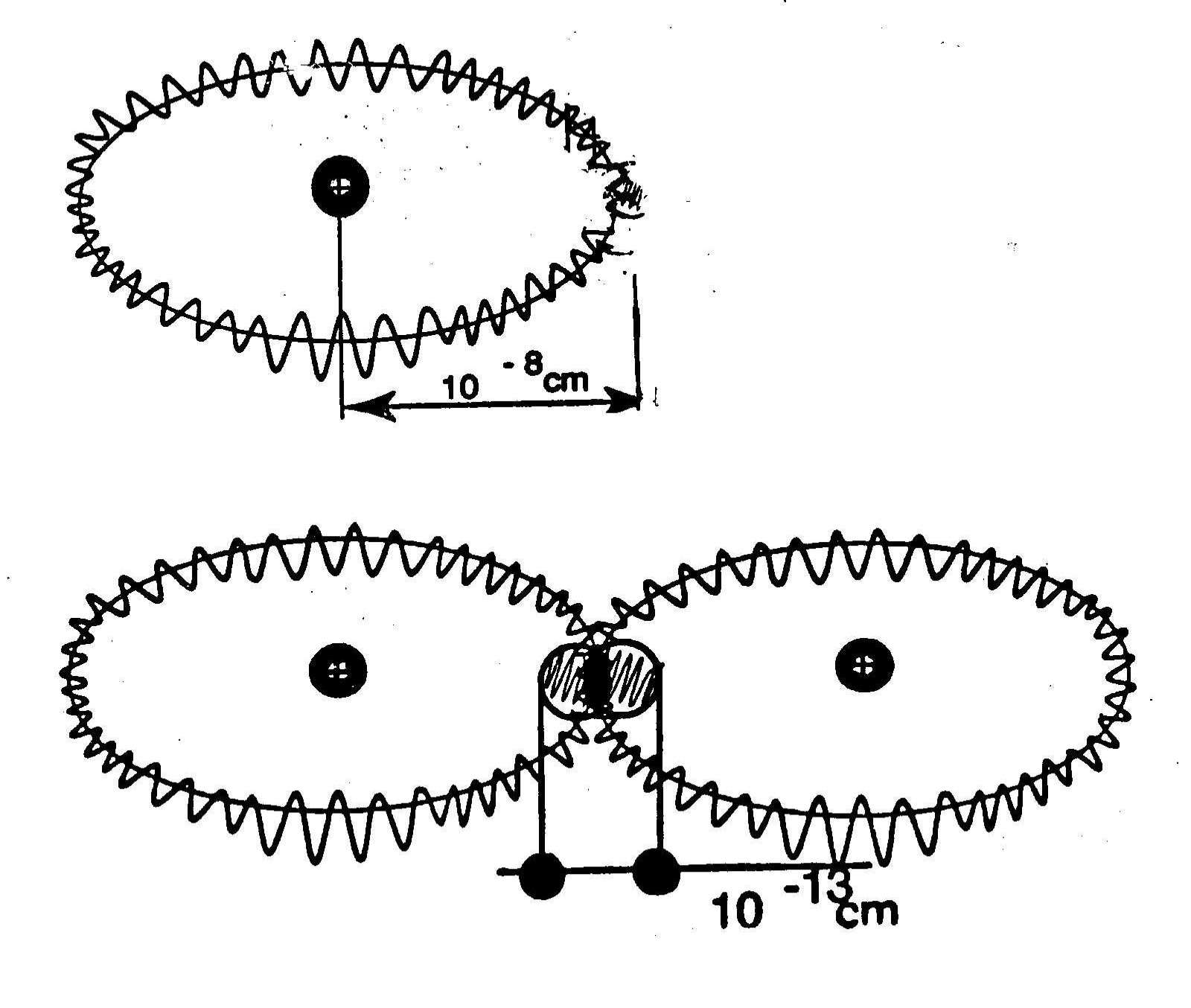
Figure 4.1. The original drawing used by Santilli to
illustrate the physical differences between the hydrogen atom and the
hydrogen molecule, the former consisting of point-like particles at
large mutual distances, and the latter having additional short range
interactions necessary for the valence bond. These physical differences
illustrate the exact character of quantum mechanics for large mutual
distances as in the hydrogen atom and its merely approximate character
for additional short distance interactions as in the hydrogen molecule.
The above insufficiency of quantum mechanics for the hydrogen molecule
was one of the motivations for the proposal by Santilli in 1978 to
construct the hadronic covering of quantum mechanics, as per the
historical paper hereon referred to as the "original proposal of 1978"
Need of subjecting to an experimental
verification the validity within a hadron of Einstein special relativity
and Pauli exclusion principle
R. M. Santilli, Hadronic J. Vol. 1, 574-901 (1978)
4.1B. Insufficiencies of the quantum chemical notion of valence
(1978)
Since nuclei do not participate in any appreciable way to molecular
bonds, it is evident that the lack of exact character of quantum
mechanics for the structure of the H-H molecule is due to the valence
bond, namely, to the appearance of interactions and effects at the
short distance conditions of the valence bond beyond the descriptive
capabilities of quantum mechanics (see Figure 4.2),
For these and other reasons, Santilli never accepted the quantum
chemical notion of valence bonds since his graduate studies in the 1960s
at the University of Torino, Italy. In particular, as indicated in
Section 1.9, Santilli always considered said notion as a pure
nomenclature without quantitative scientific content because, to
achieve the latter, the valence must verify the following:
CONDITION 1: Identify explicitly, that is, with equations, the
force between a pair of valence electrons and its physical or
chemical origin;
CONDITION 2: Prove that the above identified force is indeed
attractive; and
CONDITION 3: The above identified attractive force must verify
experimental evidence on molecular binding energies and other data.
Quantum chemistry cannot verify the above conditions because
identical electrons repel each other, and certainly they do not
attract each other, according to quantum mechanics and chemistry.
Hence, Santilli sets his research objective of building a covering of
quantum chemistry more adequate for the representation of the valence
and other chemical features.
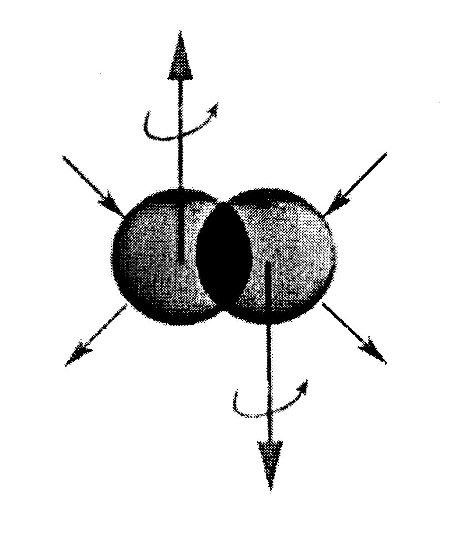
Figure 4.2. A reproduction of the original picture used by
Santilli on the valence electrons bond in single coupling illustrating
the need for a theory that is nonlinear (in the wavefunction), nonlocal
(of integral type) and nonunitary (because of contact nonpotential type
not representable with a Hamiltonian). Quantum chemistry is strictly
linear, local-differential and Hamiltonian, thus being structurally
unable to provide a quantitative representation of the valence. By
comparison, the covering hadronic chemistry has the needed nonlinear,
nonlocal and nonunitary structure beginning with its mathematical
foundation.
The insufficiency of the quantum notion of valence was the central
motivation for the construction of hadronic mechanics and chemistry, as
stressed in the Original Proposal of 1978 quoted above, to such an
extent that two intersecting circles were assumed as the first logo of
the Institute for Basic Research.
4.1C. Insufficiencies of screened Coulomb potentials (1978)
The impossibility for the conventional formulation of quantum chemistry
to provide an exact representation of molecular binding energies and
other data became clear in the second part of the 20th century. The
resolution of the insufficiencies was then attempted via the so-called
screening of the Coulomb potential, that is, the multiplication
of the fundamental Coulomb potential between two valence electrons, V =
e2/r, by an arbitrary function f(r) of completely unknown
origin, resulting in "screened potentials" of the type
(4.1) V'(r) = f(r) e2/r.
The arbitrary function f(r) was fitted from experimental data; screened
Coulomb potentials did achieve the intended accuracy in the
representation of binding energies; and quantum chemistry was confirmed
as being exactly valid for molecular structures.
Despite the above success, Santilli never accepted screened Coulomb
potentials for the following reasons:
1) .The map from the Coulomb potential to its screened form requires a
nonunitary transform
(4.2) V(r) = e2/r → V'(r) = f(r)
e2/r = U V(r) U†.
(4.3) UU† = f(dr) ≠ I .
Consequently, the screening of the Coulomb law causes major departures
from the unitary structure of quantum mechanics
2) The Coulomb potential is a fundamental invariant of quantum
mechanics. Consequently, its screening causes the breaking of the
fundamental Galilei symmetry under which conditions quantum mechanics
cannot any longer be exact.
3) It is well known that the quantum of energy is solely possible
for the Coulomb law and that any quantization of the energy is
impossible for screened potentials.
For these and other reasons, Santilli always rejected as inappropriate
the name of "quantum chemistry" for screened Coulomb potentials.
In the scientific reality, it is clear that the screening of the Coulomb
law is outside the class of unitary equivalence of quantum mechanics and
chemistry; hadronic mechanics and chemistry are indeed the broadest
possible nonunitary coverings of quantum theories; and, therefore,
screened potentials are a particular case of the nonunitary class of
interactions treated by hadronic mechanics. To put it explicitly,
Santilli showed that hadronic chemistry was already in use by the end of
the 20th century, although under the disguised name of "quantum"
chemistry.
As one can verify, Santilli's Original Proposal of 1978 was centered in
the construction of a nonunitary covering of quantum mechanics
also in view of the nonunitary character of map (4.2). Hence, the
insufficiencies of quantum chemistry had a crucial role for the
conception, development and verification of hadronic mechanics.
4.1D. Classification of hadronic chemistry (2000)
Immediately following the achievement in 1996 of mathematical maturity
of hadronic mechanics, Santilli passed to applications of the new
mechanics in chemistry because some of the most important experimental
verifications and industrial applications of the new discipline was
expected precisely in chemistry. These studies produced a covering of
quantum chemistry known as hadronic chemistry comprising the
following branches:
QUANTUM CHEMISTRY: assumed to be exactly valid for all mutual
distances of particles bigger than 1 fm = 10-13 cm;
ISOCHEMISTRY: characterized by a Lie-isotopic, time invariant,
axiom-preserving, nonunitary covering of quantum chemistry formulated
over Hilbert-Santilli isospaces over Santilli isofields for the
representation of isolated and reversible chemical structures and
processes;
GENOCHEMISTRY: characterized by a Lie-admissible, time
irreversible covering of isochemistry formulated on Hilbert-Santilli
genospaces over Santilli genofields for the representation of
irreversible chemical structures and processes;
HYPERCHEMISTRY: characterized by a multi-valued covering of
genochemistry for the representation of organic structures and
processes;
ISODUAL ISO-, GENO- AND HYPER-CHEMISTRY: characterized by the
isodual map (2.9) for the description of the chemistry of
antimatter.
4.1E. Basic literature
Hadronic mechanics achieved mathematical maturity in a special issue of
the Rendiconti Circolo Matematico Palermo of 1996 entirely
dedicated to Santilli's new mathematics, which issue also presented the
first formulation of Santilli hypermathematics needed for biology
Nonlocal-integral isotopies of
differential calculus,
mechanics and geometries
R. M. Santilli, Rendiconti Circolo Matematico Palermo, Suppl. Vol.
42, 7-82 (1996).
The main historical reference in hadronic chemistry is the 2001
monograph on Foundation of Hadronic Chemistry hereon refereed to
as FHC:
"Foundations of Hadronic Chemistry,
with Applications to New Clean Energies and Fuels"
R. M. Santilli,
Kluwer Academic Publishers (2001)
Subsequent studies can be found in Hadronic Mathematics Mechanics
and Chemistry, hereon referred to as HMMC Volumes I, II, III, IV and
V:
Hadronic
Mathematics, Mechanics and Chemistry, Volumes I, II, III, IV and V:
R. M. Santilli, International Academic Press (2008)
Santilli's discovery of new magnecular fuels with complete combustion,
and related industrial realization, can be found in the monograph
The novel magnecular species of hydrogen and oxygen
with increased specific weight and energy content
R. M. Santilli,
Intern. J. Hydrogen Energy Vol. 28, 177-196 (2003)
A general review of the new species of magnecules is available from the monograph
The New Fuels with Magnecular Structure
Ruggero Maria Santilli
International Academic Press (2008)
200 pages
Italian translation by Giovanna Bonfanti and Michele Sacerdoti,
published by Editori Riuniti, Roma, Italy, and available at
I Nuovi Carburanti con Struttura Magnecolare
The main references on Santilli's studies in biology can be found in the
monographs
"Isotopic, Genotopic and Hyperstructural Methods in Theoretical
Biology"
R. M. Santilli,
Ukraine Academy of Sciences (1997)
The Australian biologist Chris R. Illert provided important input in
Santilli's studies in biology, on which we quote the joint monograph
"Foundation of Theoretical Conchology"
C. R. Illert and R. M. Santilli,
Hadronic Press (1995)
Various, additional, specialized papers will be identified during the
course of our presentation.
It should be stressed that in this chapiter we shall present for
notational simplicity only the projection of Santilli's hadronic
chemistry on a conventional Hilbert space over a conventional field, so
as to avoid the complex notations of the full hadronic treatment. The
understanding is that only the latter treatment resolves the Theorems of
Catastrophic Inconsistencies of Section 3.7. Therefore, readers without
a technical knowledge of hadronic mechanics are suggested to abstain
from venturing judgments on the content of this chapter so as to avoid a
clear illusion of knowledge.
4.2. HADRONIC CHEMISTRY
4.2A. Animalu-Santilli Cooper pair (1995)
The application and experimental verification of hadronic mechanics
that anticipated hadronic chemistry is given by the first quantitative
representation in history of the structure of the Cooper pair in
superconductivity. As it is well known, quantum mechanics does provide a
consistent representation of superconductivity, but via an
ensemble of Cooper pairs considered point-like, without any
description on how identical electrons could bond themselves into the
Cooper pair, since electrons repel each other according to quantum
mechanics.
A dominant model in the quantum literature in the field is that based on
the interplay of entities called "phonons" that, however, are known to
have a purely mathematical character since no "phonon" has ever been
discovered, or can at least be formulated, within the context of
elementary particle physics.
Independently from this basic insufficiency, the quantum description
of superconductivity has long surpassed the boundaries of quantitative
predictions for the increase of superconductive temperatures, recent
efforts being essentially conducted on grounds of trials and errors
without any mathematical and/or physical and/or chemical guiding
foundations.
Hadronic mechanics permitted the first quantitative representation of
the structure of the Cooper pair without any use of hypothetical
"phonons", in a way fully compatible with available experimental data, as
well with remarkable predictive capacity for bigger superconductive
temperature.
The origin of the bond between the identical electrons of the Cooper
pair resulted to be the contact nonpotential interactions
occurring in the deep mutual penetration and overlapping of the
wavepackets of the electrons, as first identified by Santilli in the
Original Proposal of 1978. The trigger for the bond of the electrons
resulted to be due to the cuprate as well as other nuclei. The
nonpotential character of the interactions rendered mandatory the use of
the sole invariant mechanics for their representation, hadronic
mechanics.
In this way, the hypothetical "phonons" providing a hypothetical
exchange bond between electrons that repel each other according to
quantum laws, resulted as being a mere mathematical mechanism for the
approximation of contact nonpotential interactions beyond any
capability of quantum representations. The main gain in the process is a
dramatic increase of the predictive capacities to increase the
superconductive temperature, all the way to the prediction of a new
electric current based on the transfer of Cooper pairs or, more
appropriately, valence electrons pairs in singlet bond, since the latter
have no appreciable magnetic moment, with evident dramatic decrease of
the resistance.
Among a large number of publications in the field, we quote the
historical paper by A. O. E. Animalu and R. M. Santilli of 1995
Nonlocal isotopic representation of the
Cooper pair in superconductivity
A. O. E. Animalu and R. M. santilli,
Intern. J. Quantum Chemistry Vol. 29, 175-187 (1995)
Vast studies in the field were conducted by Animalu and reviewed in
details in HMMC, Volume V, where Santilli gave the name of Animalu
isosuperconductivity to the resulting new discipline.
4.2B. Santilli-Shillady strong valence bond (1999)
The central problem of molecular chemistry is the verification of
Conditions 1, 2, 3 of Section 4.1. This objective was achieved by R. M.
Santilli and the American chemist D. D. Shillady in the historical paper
of 1999 .
A new isochemical model of the hydrogen molecule
R. M. Santilli and D. D. Shillady,
Intern. J. Hydrogen Energy Vol. 24, pages 943-956 (1999)
To provide a conceptual outline, consider the conventional quantum
mechanical equation in relative coordinates and reduced mass for two
electrons in singlet coupling as per Figure 4.2,
(4.4) (p2/m + e2/r) ψ(r) = E
ψ(r),
where m is the electron mass. The above equation shows the
repulsive Coulomb force between the point-like charges of
the electrons. But the electrons have extended wavepackets of
the order of 1 fm whose mutual penetration, as necessary for the valence
bond, causes nonlinear, nonlocal and nonpotential interactions
at the foundations of hadronic mechanics (see the preceding chapter).
The only known possibility fo an invariant representation of these
interactions is to exit from the class of unitary equivalence of Eq.
(4.4) via an isounitary transformation (see Section 3.11) that, for
simplicity, we present below in its projection into a
conventional nonunitary form
(4.5) UU† ≠ I, UU† =
I* = 1/T ,
(4.6) U [(p2/m + e2/r) ψ(r)]
U† =
= [ (Up2U†)/m +
(e2/r)UU†]
(UU†)-1 [Uψ(r)U†]
=
= [1/m)p* T p* T + e2/r ]
ψ*(r) = E' ψ*(r),
where one should note the different eigenvalue E' from the value E of
Eq. (4.4) (due to the general noncommutativity of the Hamiltonian and
the isounit).
At this point, Santilli and Shillady introduce the following realization
of the nonunitary transform, that is, of the fundamental isounit of
hadronic chemistry, Eq. (4.7) of FHC,
(4.7) UU† = I* = 1/T =
= e{[ψ(r)/ψ*(r)] ∫
ψ†1(r) ψ2(r)
d3(r)} = 1 + [ψ(r)/ψ*(r)] ∫
ψ†1(r) ψ2(r)
d3(r) + ...
where ψ and ψ* are the solutions of the unitary and
nonunitary equations, and ψk, k = 1,2, are the
conventional quantum mechanical wavefunctions of the two electrons.
It is evident that, as desired, the above isounit represents
interactions that are: nonlinear, because dependent in a nonlinear way
in the wavefunctions; nonlocal, because inclusive of a volume integral;
and nonpotential, because not representable with a Hamiltonian.
Additionally, for all mutual distances between the valence electrons
greater than 1 fm, the volume integral of Eq. (4.7) is null with the
crucial limit
(4.8) Limr bigger 1 fm I* = 1,
under which hadronic chemistry recovers quantum chemistry identically
and uniquely. As it was the case for hadronic mechanics, hadronic
chemistry merely provides a form of "completion" of quantum chemistry at
short distances much along the celebrated intuition by Einstein,
Podolsky and Rosen.
Santilli and Shillady solved the above equations in all details. First,
by inserting isounit (4.7) in Eqs. (4.6), they obtained the
isoequation here projected on a conventional Hilbert space
(4.9) [p2/2m' + e2/r -
Voe-br/(1 - e-br)] ψ*(r)
= E'ψ*(r),
where m' represents the isorenormalization of the mass caused by
nonpotential interactions, and one recognizes the emergence of the
attractive Hulten potential
(4.10) VHulten = Voe-br/(1 -
e-br).
But the Hulten potential is known to behave like the Coulomb potential
at short distances and be much stronger than the latter. Therefore, Eq.
(4.9) admits the excellent approximation
(4.11) [p2/2m' - V' e-br/(1 -
e-br)] ψ*(r) = E'
ψ*(r),
where the new constants V' reflects the "absorption" of the repulsive
Coulomb potential by the much stronger attractive Hulten
potential.
In this way, Santilli and Shillady achieved for the first time in the
history of chemistry a valence coupling between two identical electrons
in singlet coupling with a strongly attractive force, as requested by
experimental evidence, which model is today known as the
Santilli-Shillady strong valence bond.
4.2C.The isoelectronium (1999)
Another major insufficiency of quantum chemistry is the lack of
restriction of the valence correlation-bond specifically and solely to a
valence pair. This additional insufficiency causes further
inconsistencies between the prediction of the theory and reality, such
as the prediction that all molecules are paramagnetic, in dramatic
disagreement with experimental evidence.
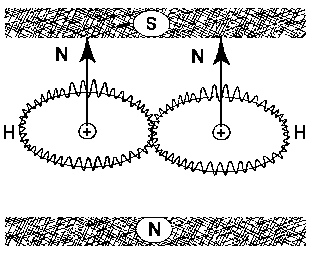
Figure 4.3. The original drawing used by Santilli to
illustrate a catastrophic consequence of the quantum chemical notion of
valence, the prediction of the paramagnetic character of all substances.
The prediction includes the hydrogen molecule, in dramatic
disagreement with experimental evidence according to which the hydrogen
molecule is diamagnetic, thus being unable to acquire a total magnetic
polarization under an external magnetic field. The indicated prediction
is a consequence of the absence in quantum chemistry of a strongly
attractive valence force, in which case valence electrons are
unconstrained and, consequently, can acquire independent magnetic
polarizations under an external magnetic fieod. The picture provides a
conceptual rendering of the two H-atoms of the hydrogen molecule as
essentially being independent. The alternative conception of orbitals
distributed around the two nuclei carries an even stronger prediction of
paramagnetic character because it can acquire more easily a total
polarization under an external magnetic field contrary to nature.
Alternatively and equivalently, the fact that the hydrogen molecule is
not paramagnetic can solely be represented via a strong bond between a
valence electron pair into a quasiparticle Santilli and Shillady called
the isoelectronium, that, as a necessary condition to avoid the
prediction of the paramagnetic character of the H-H molecule, must have
an oo-shapes orbit around the individual H atom as shown in Figure 4.4.
In the event the isoelectronium orbits along a single external orbit
encompassing the two nuclei, its orientation under a sufficiently strong
external field is consequential, resulting again in the catastrophic
prediction of the paramagnetic character of the H-H molecule.
On technical grounds, the isoelectronium is the bound state of two
identical valence electrons in singlet coupling characterized by the
isoequation (4.9). Intriguingly, said equation admitted one and only one
eigenvalue, thus characterizing the isoelectronium as a quasiparticle
possessing the following main characteristics (see FHC for details):
ISOELECTRONIUM: Mass = 1.022 MeV; spin 0; charge 2e; magnetic
moment 0; radius b-1 = 6.8432 10-11 cm.
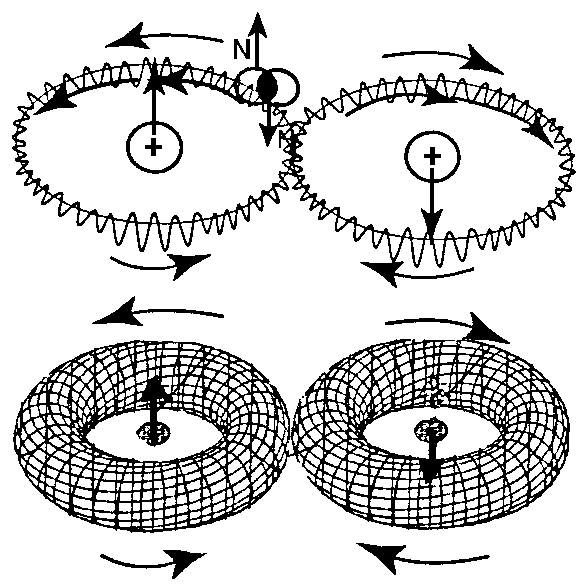
Figure 4.4. A view of isochemical model of the hydrogen
molecule at absolute zero degrees temperature, thus without any
rotational degree of freedom, with the Santilli-Shillady strong valence
bond between valence electrons pairs into the isoelectronium
quasiparticle. Note the oo-shaped orbital of the isoelectronium, the
only one allowing a representation of the diamagnetic character since,
under an external strong magnetic field, the two H atoms acquire
parallel but opposite magnetic polarities with null value at sufficient
distance. Note also the toroidal distribution of the orbital of the
isoelectronium due to the isouncertainty principle of hadronic
mechanics,
Needless to say, the isoelectronium is unstable because the
isouncertanty principle predicts the tunneling of the electrons through
the nonpotential barrier. However, the bond between identical electrons
in singlet coupling is so strong to cause the recombination of the
isoelectronium following its spontaneous disintegration since the bond
is attractive at distances much bigger than b-1 due to the
extended character of the wavepackets.
It should be indicated that the name "isoelectronium" was proposed to
emphasize the fact hat the individual electrons in valence couplings
are not conventional particles, but isoelectrons, that is, electrons
under Hamiltonian and non-Hamiltonian interactions characterized by the
fundamental Poincare'-Santilli isosymmetry, thus having not only
conventional renormalizations of kinematical characteristics
caused by Hamiltonian interactions, but also isorenormalizations of
intrinsic characteristics caused by non-Hamiltonian interactions.
A fundamental feature is that the strongly attractive
Santilli-Shillady valence bond has no potential energy by central
assumption because originating from nonpotential/non-Hamiltonian
interactions. By recalling that the mass of the electron is 0.511
MeV, the mass of the isoelectronium 1.022 MeV is therefore assumed under
the assumption that the positive potential energy for the Coulomb
repulsion cancels out with the negative binding energy of the electron
magnetic fields that, in singlet coupling, is attractive.
4.2D. The hydrogen molecule (1999)
By combining the vast advances outlined until now, hadronic chemistry
permitted the first achievement of an exact-numerical representation of
all characteristics of the hydrogen molecule H2 = H-H from
unadulterated first principles without ad hoc adulterations, including
binding energy, electric and magnetic moments, size, and other
features, as presented in FHC Section 4.4 and the historical paper
A new isochemical model of the hydrogen molecule
R. M. Santilli and D. D. Shillady,
Intern. J. Hydrogen Energy Vol. 24, pages 943-956 (1999)
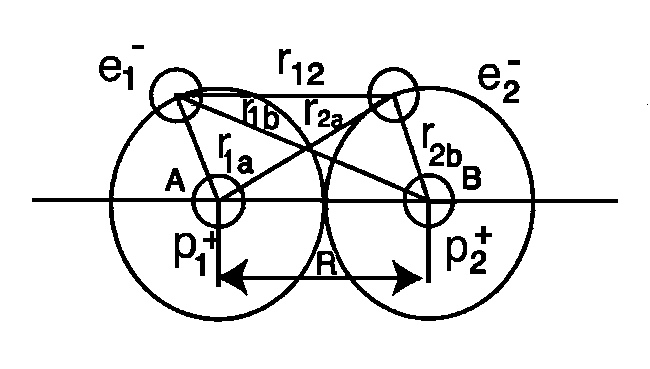
Figure 4.5. A view of all possible conventional interactions
between the two electrons and the two protons of a hydrogen molecules.
Being quantum mechanical, all these interactions are solely at a
distance derivable from a potential, as in Eq. (4.12), thus being
basically insufficient for a representation of the short term
nonpotential interactions in valence bonds.
As it is well known, according to quantum chemistry, the hydrogen
molecule is a four body system comprising two protons and two
electrons with conventional equation for the molecule at rest, that is,
the two protons are considered as rest as conventionally done
(4.12) [p12/2m1 +
p22/2m2 + e2/r12
- e2/r1a - e2/r1b -
e2/r2a - e2/r2b +
e2/R] ψ(r) = E ψ(r),
where: 1, 2 represents the two electrons; a, b represent the two
protons; and R is the distance between the protons. Due to its four-body
character, the above equation, does not admit any analytic solution;
misses at least 2% of the binding energy; and predicts that the hydrogen
molecule is paramagnetic due to the evidence independence of the
electrons.
The repetition of the nonunitary transform of the preceding section
yield the isochemical model of the hydrogen molecule as a four-body
system
(4.13) [p12/2m'1 +
p22/2m'2 - V*
e-br/(1 - e-br) + e2/R]
ψ*(r) = E' ψ*(r),
showing the appearance of the Santilli-Shillady strong valence bond of
Hulten type that "absorbs" all coulomb potentials, where m' represents
the isorenormalized mass of the electrons.
In the figures below we provide the results of the solutions of the
isochemical model (4.13) achieved via variational methods by Santilli
and Shillady in their historical paper of 1999, with detailed
elaboration additionally provided in FHC, Chapter 4.
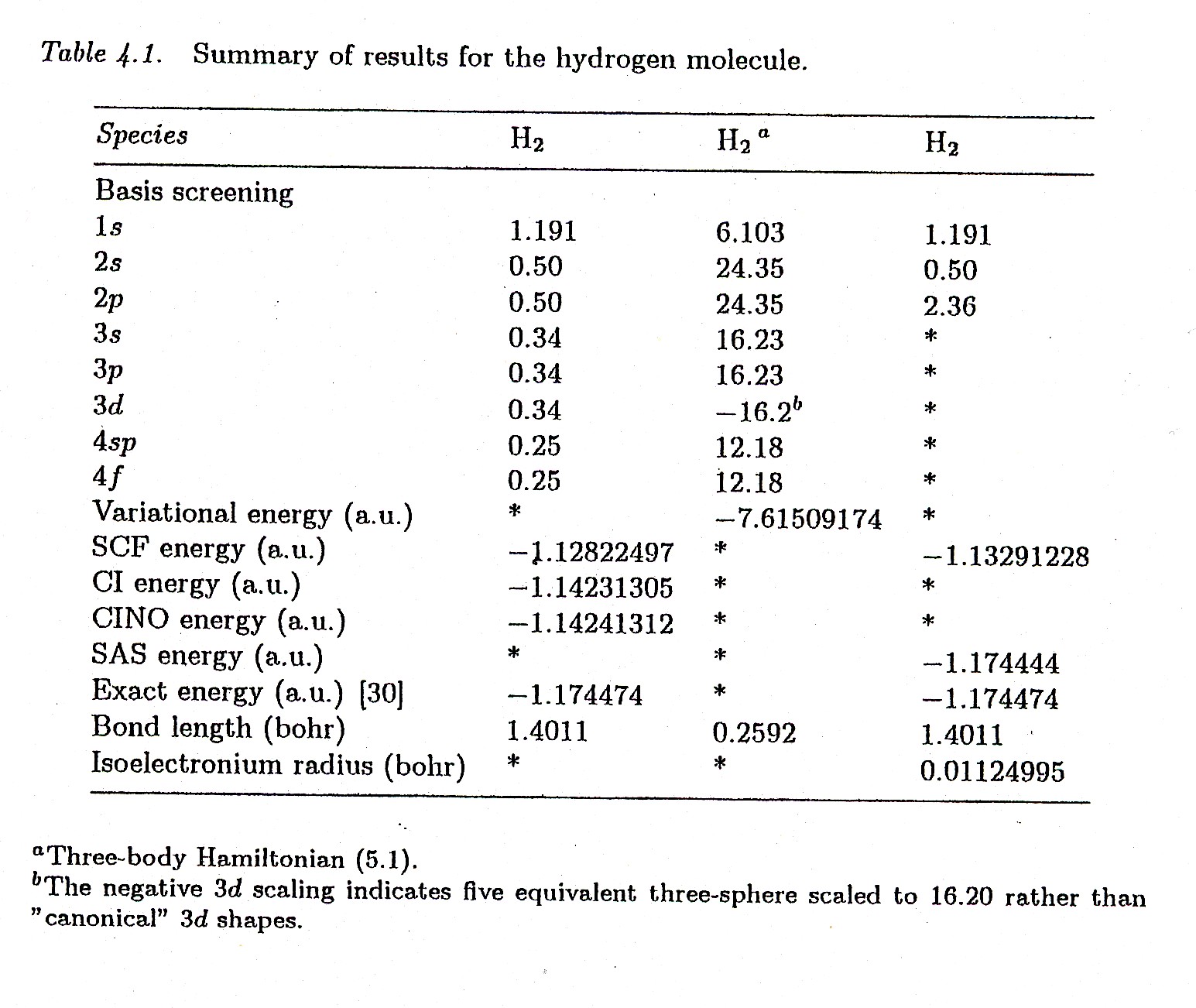
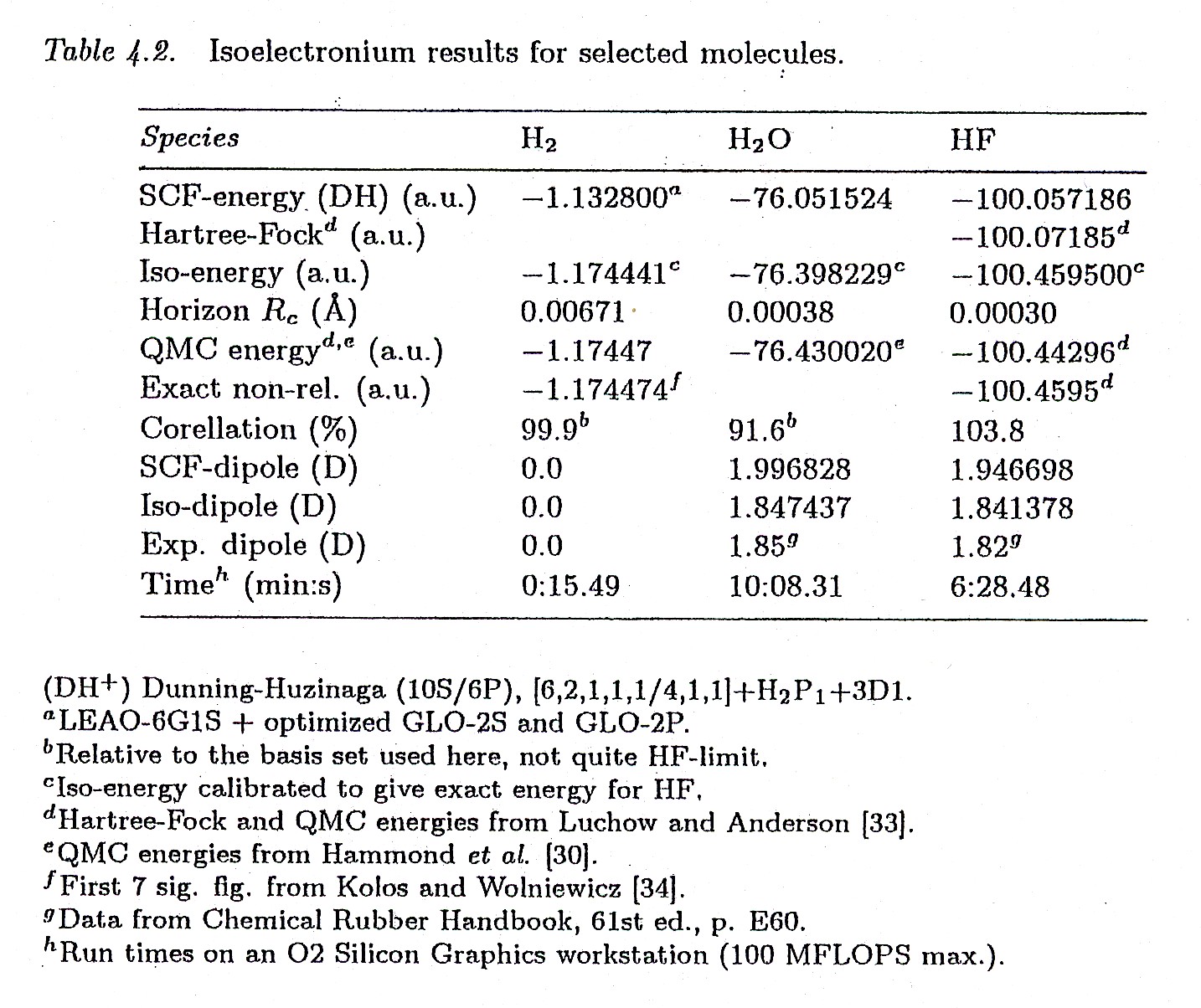
Figure 4.6. A reproduction of Tables 4.1 and 4.2 of
Santilli's FHC showing the achievement by isochemistry of a numerically
exact representation of all features of the hydrogen molecule.
However, a fundamental implication of hadronic chemistry is that of
restricting the above four-body model to a three-body structure
evidently composed by the two protons at mutual distance R and the two
valence electrons strongly bonded into the isoelectronium quasiparticle.
In fact, by repeating the nonunitarity map of the preceding section,
the isochemical model of the hydrogen molecule as a three-body
system can be written
(4.14) [pisoel2/2misoel +
pa2/2mprot +
pb2/2mprot - V*
e-br/(1 - e-br) + e2/R] ψ(r) = E'
ψ(r),
where: the system does admit an analytic solution in its restricted form
under the assumption that the isoelectronium is stable; and one should
note again the change of the eigenvalue in the transition from Eq.
(4.12). Note also that Eq. (4.14) is purely quantum chemical because all
distances between the constituents are much bigger than 1 fm.
An exact variational solution of model (4.14) was first studied
in 2000 by A.K. Aringazin and M.G. Kucherenko in the paper
Exact variational solution of the restricted three-body
Santilli-Shillady model of the hydrogen molecule
A.K. Aringazin and M.G. Kucherenko,
Hadronic J. Vol. 23, 1-56 (2000) (physics/0001056)
The exact analytic solution of model (4.14) was achieved in 2007
by R. Perez-Enriquez and R. Riera in the paper
Exact analytic solution of the restricted three-body Santilli-Shillady
model of the hydrogen molecule
R. Perez-Enriquez and R. Riera,
Progress in Physics Vol. 2, 34-41 (2007) (physics/0001056)
4.2E. The water molecule (2000)
Despite a deceptive simplicity, the water molecule H2O =
H-O-H is one of the most complex structures in nature whose
understanding, let alone its representation, is beyond the capability of
the rather limited theories of the 20th century because of the following
additional insufficiencies or inconsistencies:
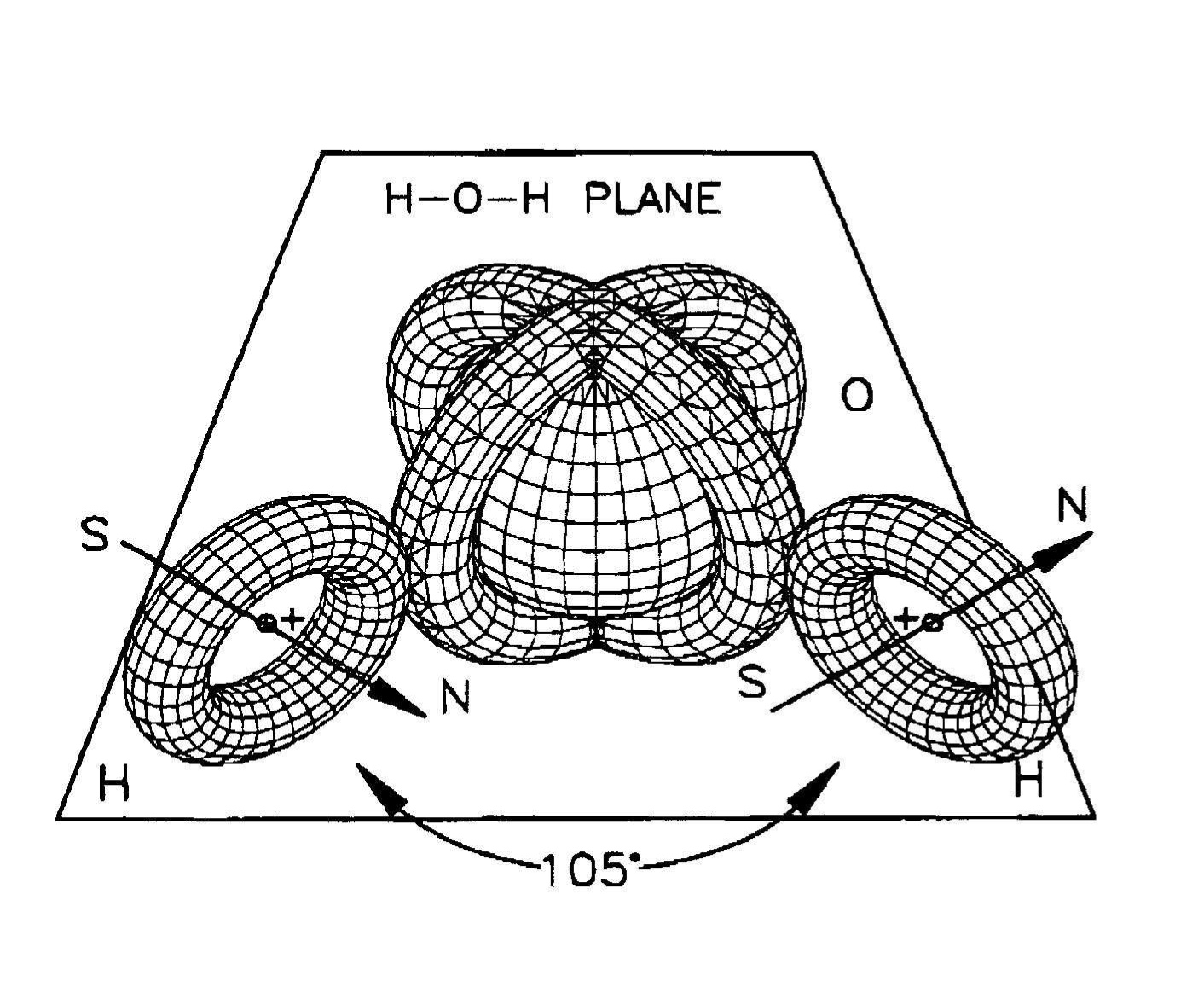
Figure 4.7. A view of the water molecule H2O at
absolute zero degree temperature, thus without any rotational degree of
freedom, showing the H-O-H plane, the 105o between the H-O
and O-H dimers and, above all, the natural occurrence according to which
the orbitals of the H atoms are not spherical, but of toroidal character
for their coupling with the oxygen, thus providing a direct verification
of the isochemical model of the hydrogen molecule of Figure
4.5
1) Quantum chemistry not only fails to achieve an exact representation
of the binding energy of the water molecule from first principles
without ad hoc adulterations, but predicts electric and magnetic
polarizations that are wrong even in the sign, let alone in their
(absolute) values.
2) Quantum chemistry is a structurally linear theory, thus representing
complex multi-body systems such as the water molecule via the
factorization of the total wavefunction into its individual components,
(4.15) ψtotal = ψ1
ψ2 ... ψn
which factorization, in turn, requires the validity of the
superposition principle as a pre-requisite for consistency.
However, multi-body systems are nonlinear (in the wavefunction and
other quantities), under which occurrence the superposition principle is
inapplicable, with consequential impossibility of formulating a
consistent factorization
(4.16) H(r, p, ψ, ...) ψtotal ≠ H(r, p,
ψ, , ...) ψ1 x ψ2 x ...
ψn.
thus resulting in the inapplicability of the very axioms of quantum
chemistry to complex structures such as the water molecule.
3) When passing to the synthesis of the water molecule, the
insufficiencies of quantum chemistry become embarrassing because the
former process is structurally irreversible, while the latter theory is
reversible, thus predicting with equal statistical probability both the
synthesis and its spontaneous decay (Section 1.9)
(4.17) H2 + O → H2O
(4.18) H2O → H2 + O.
thus becoming inapplicable for a serious study.
4) When passing to water molecules as part of complex environments such
as in the liquid state or when at the foundation of life, the
insufficiencies of quantum chemistry become simply beyond any level of
acceptability.
5) Besides all these limitations or sheer inconsistencies, quantum
chemistry admits an additional rather crucial limitation given by the
poor convergence of perturbative series. In essence, the water and other
complex molecules are multi-body systems, thus requiring approximate
solutions via variational, perturbative, Gaussian, and other methods,
all based on expansions whose calculations require computers due to
their complexity. The insufficiency here referred to is given by the
fact that the time requested for basic calculations by large computers
is generally excessive, thus implying an inherent lack of strong
convergence of the underlying perturbative series, with consequential
debatable accuracy.
All the above insufficiencies or sheer inconsistencies establish that
the selection of the appropriate generalization of quantum
chemistry should indeed be subjected to scientific debate, but its
denial for the preservation of an old theory is equivocal and
ascientific.
Santilli conceived and constructed hadronic mechanics and chemistry for
the solution of the above insufficiencies. In fact:
1*) Hadronic chemistry achieved the first exact
representation of all features of the water molecule from first
unadulterated principles;
2*) The reconstruction of linearity on the Hilbert-Santilli
isospace over Santilli isofields (isolinearity) achieved via the
embedding of all nonlinear terms in the isounit and isotopic element,
(4.19) H(r, p, ψ, ...) = Ho(r, p)
T(ψ,...),
and restores the superposition principle with exact factorization
(4.20) Ho(r, p) T(ψ, ...) ψtotal = Ho T(r, p, ψ, , ...)
ψ1 x ψ2 x ... ψn.
with consequential validity of the isotopic theory for complex systems.
3*) The entire branch of genochemistry has been
constructed by Santilli with an irreversible structure precisely to
resolve the inconsistency 3).
4*) The additional branch of hyperchemistry has been
built by Santilli precisely to initiate the study of complex inorganic
and organic structures, as we shall see later on this chapter (see
Section 4.5 below).
5*) Yet another central feature of isochemistry is that the
absolute value of the isounit, such as Eq. (4.7), is much bigger than
one and the isotopic element is much smaller than one. Consequently, all
series that are slowly convergent are turned into a strongly (fast)
convergent form. In fact, the variational calculations for the
isochemical model of the water molecule turned out to require a
computer time at least 1,000 shorter than that of conventional
calculations (see FHC for details).
The understanding of hadronic chemistry requires the knowledge that it
permits a series of treatments of the water molecule with increasing
complexities and methodological capabilities. The first treatment
addressed in this section is that via isochemistry and applies when the
water molecule is assumed as isolated from the rest of the universe,
reversible over time and at absolute zero degrees temperature, thus
without any rotational degrees of freedom as in Figure 4.7.
ISOCHEMICAL MODEL OF THE WATER MOLECULE:
The model achieved for the first time the exact representation from
first axiomatic principles without ad hoc adulterations of the binding
energy, sign and values of the electric and magnetic moments, and other
features as first presented by Santilli and Shillady in their second
historical paper of 2000
A new isochemical model of the water molecule
R. M. Santilli and D. D. Shillady,
Intern. J. Hydrogen Energy Vol. 25, 173-183 (2000)
with a detailed presentation available in FHC, Chapter 5. The study is
too complex for a technical outline in this section. Therefore, we limit
ourselves to the following conceptual summary.
The model can be constructed and worked out via a series of
progressively improving representations. The simplest one is given by
achieving an exact solution for the dimer O-H and then introducing the
additional H atom as a perturbation. This can be done by representing
the oxygen as a two-body ion O- characterized by one valence
electron and all the rest of the atom assumed as concentrated in its
nucleus with one single positive elementary charge.
The Santilli-Shillady strong bond of the valence electrons into the
isoelectronium then renders the system H-O- a restricted
three-body system with an exact solution. The additional H atom is then
added as a perturbation or via other mean.
By denoting with the subindics 1 and a the hydrogen and 2 and b the
oxygen, the conventional quantum chemical representation of the above
indicated H-O- dimer is similar to that of Eq. (4.12), i.e.,
(4.21) [p12/2m1 +
p22/2m2 + e2/r12
- e2/r1a - e2/r1b -
e2/r2a - e2/r2b +
e2/R] ψ(r) = E ψ(r),
Santilli and Shillady then apply a nonunitary transform as for the
hydrogen molecule, resulting in the appearance of the strongly
attractive Hulten potential as in model (4.13). At this point, the bond
of the second H atom can be represented via a nonunitary image of the
Coulomb law resulting in screening of Gaussian type
(4.22) 2e2/r → 2e2(1 +/-
e-αr)/r
where: the double value 2e originate from the duality of the bonds in
H-O-H; α is a positive constant to be determined from the data;
the sign "-" applies for an O-atom as seen from an H-electron; and the
sign "+" applies for the O-atom as seen from the H-nucleus.
Note that lifting (4.22) would be aprioristic and without axiomatic
foundation for quantum chemistry, since it requires a nonunitary
image of the Coulomb law, while for the covering hadronic chemistry it
is derived from first principles, namely, via precisely the needed
nonunitary transform of quantum settings.
The implementation of the above features then yields the isochemical
model of the water molecule in its projection in the conventional
Hilbert space over conventional fields
(4.23) [p12/2m'1 +
p22/2m'2 - V*
e-br/(1 - e-br)
- 2e2/r2a - 2e2(1 - e
- αr1b)/r1b
+ e2(1 + e-αR)/R] ψ*(r) = E'
ψ*(r),
where; E' is half of the binding energy of the water molecule; R is the
interatomic distance; and the size of the isoelectronium should also be
fitted from the data.
Under the above approximation, plus the assumption that the
isoelectronium is stable, model (4.23) constitutes the first exactly
model of the water molecule from first principles in scientific
history. Such an exact solution was first reached by Santilli and
Shillady via variational methods in their historical paper of 2000, with
comprehensive variational studies conducted by A. K. Aringazin a
reproduced in Chapter 6 of FHC.
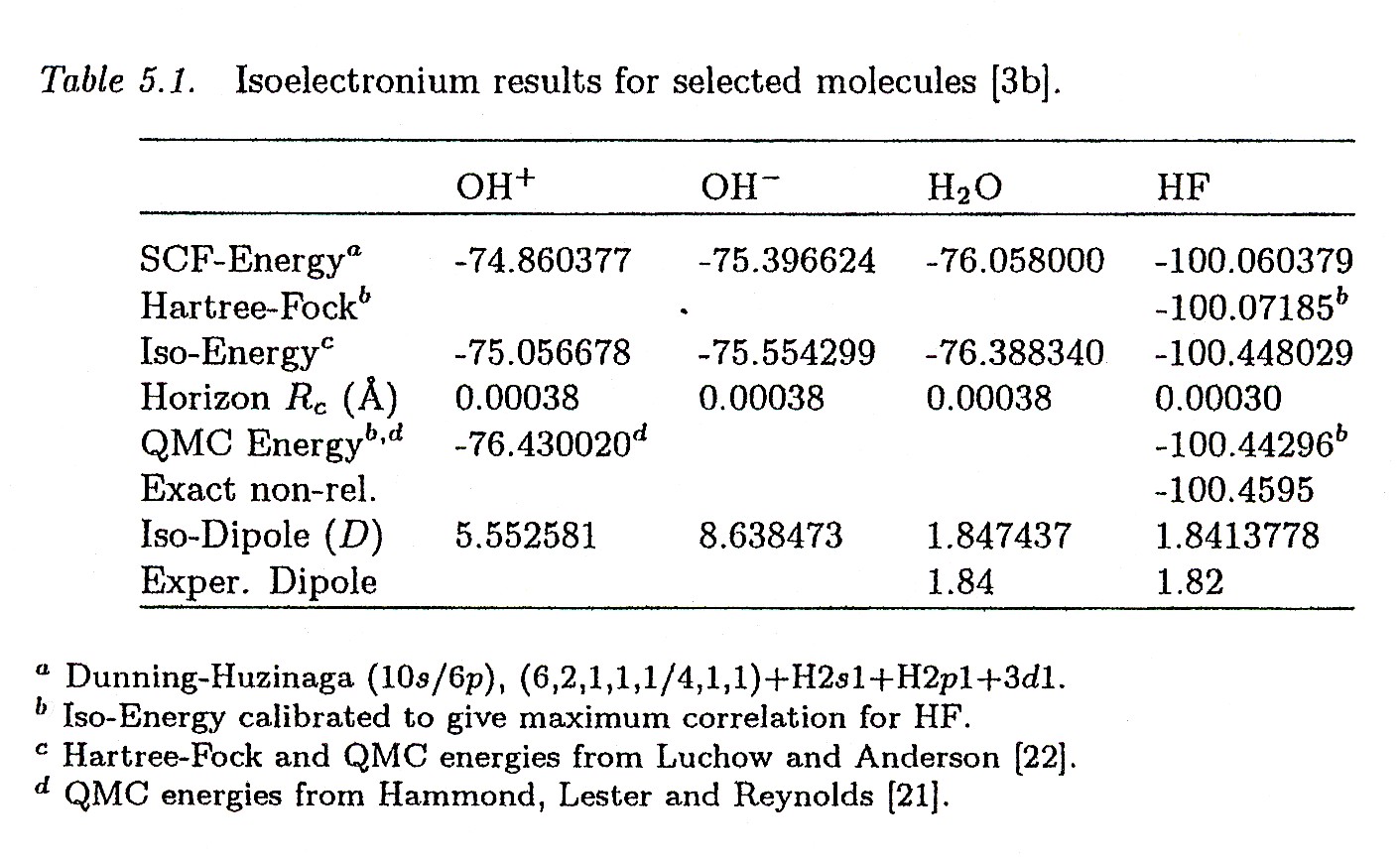
Figure 4.8. A reproduction of Table 5-1 of Santilli's FHC
summarizing the achievement of the first numerically exact
representation of the binding energy, electric and magnetic moments of
the water molecule.
Note the double nonunitary transform requested by the model, the
first for the isoelectronium and related strong bond, and the second for
the representation of the second H atom via a perturbation-screening of
the H-O dimer. Note also that the model is invariant if and only if
written on Hilbert-Santilli isospaces over Santilli isofields. Note
finally that the computer usage needed for the variational calculations
resulted to require 1/1000 shorter time than that needed for
conventional model because both unitary transforms verify the conditions
that the related isotopic element is must smaller than one.
A more accurate isochemical model of the water molecule is given by a
five body system comprising the two H nuclei, the O atom assumed
with all electrons concentrated in the nucleus except for the two
valence electrons, and two isoelectronia assumed as fully stable.
Needless to say, this model admits no analytic solution, thus requiring
variational or other approaches.
A further isochemical model is the preceding one in which the two
isoelectronia are assumed as being unstable and their meanlives are
computed from the experimental data.
GENOCHEMICAL MODEL OF THE WATER MOLECULE. It is based on the
assumption of two nonunitary transforms for the characterization
of the forward "f" and backward "b" genounits and related motion in time
(4.24) UW† = If,
WU† = bI,
(4.25) If =
(bI)†,
The model is particularly useful for the irreversible representation
of the synthesis of the water molecule H2 + O →
H2O in such a way to prevent the existence of a finite
probability for the spontaneous time reversal image.
HYPERCHEMICAL MODEL OF THE WATER MOLECULE. It is essentially
given by a multi-valued extension of the preceding models and has
resulted as having basic relevance for the initiation of the
understanding of the complexity of the water molecule when part of
living cell, thanks also to the availability of a virtually unlimited
degree of freedom for biological correlations and other complex events
(see Section 4.5 below). Note that the water molecule acquires such a
complexity as being beyond our conceptual understanding.
ISODUAL ISO-, GENO-, AND HYPER-CHEMICAL MODELS OF THE WATER
MOLECULE. They are the images of the preceding models characterized
by the isodual map (2.9,) and are used for quantitative studies of the
antimatter water molecule (see FHC for details).
Additional very important studies on hadronic chemistry were conducted
by A. K. Aringazin and his group in Kazakhstan. Besides the detailed
review of these studies by Santilli in FHC, we indicate here the
additional papers
On a variational solution of the four-body
Santilli-Shillady model of the hydrogen molecule
A.K. Aringazin, Hadronic J. Vol. 23, 57-113 (2000), physics/0001057.
Isoelectronium correlations as a nonlinear
two-dimensional two-particles tunnel effect
A.K. Aringazin and M.B. Semenov, Hadronic Journal Vol. 23, 25-53 (2000).
4.3. THE NEW CHEMICAL SPECIES OF SANTILLI MAGNECULES
4.3A. Historical notes
The dimension of Santilli's scientific conceptions can be understood by
noting that all the preceding advances on conventional molecular
structures were done as a mere preparatory basis for the
conception, quantitative treatment, experimental verification and
industrial development of a basically new chemical species, that
is, a species whose bond is NOT that of valence. The results were
presented in yet another historical paper of 1998
Theoretical prediction and experimental verification
of the new chemical species of magnecules,
R. M. Santilli,
Hadronic J. Vol. 21, 789-894 (1998)
with a detailed presentation in FHC and an update in the monograph of
2008
The New Fuels with Magnecular Structure
Ruggero Maria Santilli
International Academic Press (2008)
200 pages
Italian translation by Giovanna Bonfanti and Michele Sacerdoti,
published by Editori Riuniti, Roma, Italy, and available at
I Nuovi Carburanti con Struttura Magnecolare
plus various papers quoted in the subsequent sections.
4.3B. Conception of Santilli magnecules (1998)
The primary origin of pollutants contained in fossil fuel exhaust is the
valence bond that is so strong to prevent full combustion. Consequently,
Santilli set his research goal to search for a new way of bonding
together into stable clusters the same atoms composing fossil fuels
under the following:
CONDITION 1: The new bond should be weaker than the
valence bond as a necessary condition to decrease pollutants;
CONDITION 2: The new weaker bond should allow the formation of
clusters that are stable at industrially used storage values of
temperature and pressure, e.g., those for methane; and
CONDITION 3: The new, weaker and stable bond should
decompose itself at the combustion temperature to optimize the
energy released by the combustion.
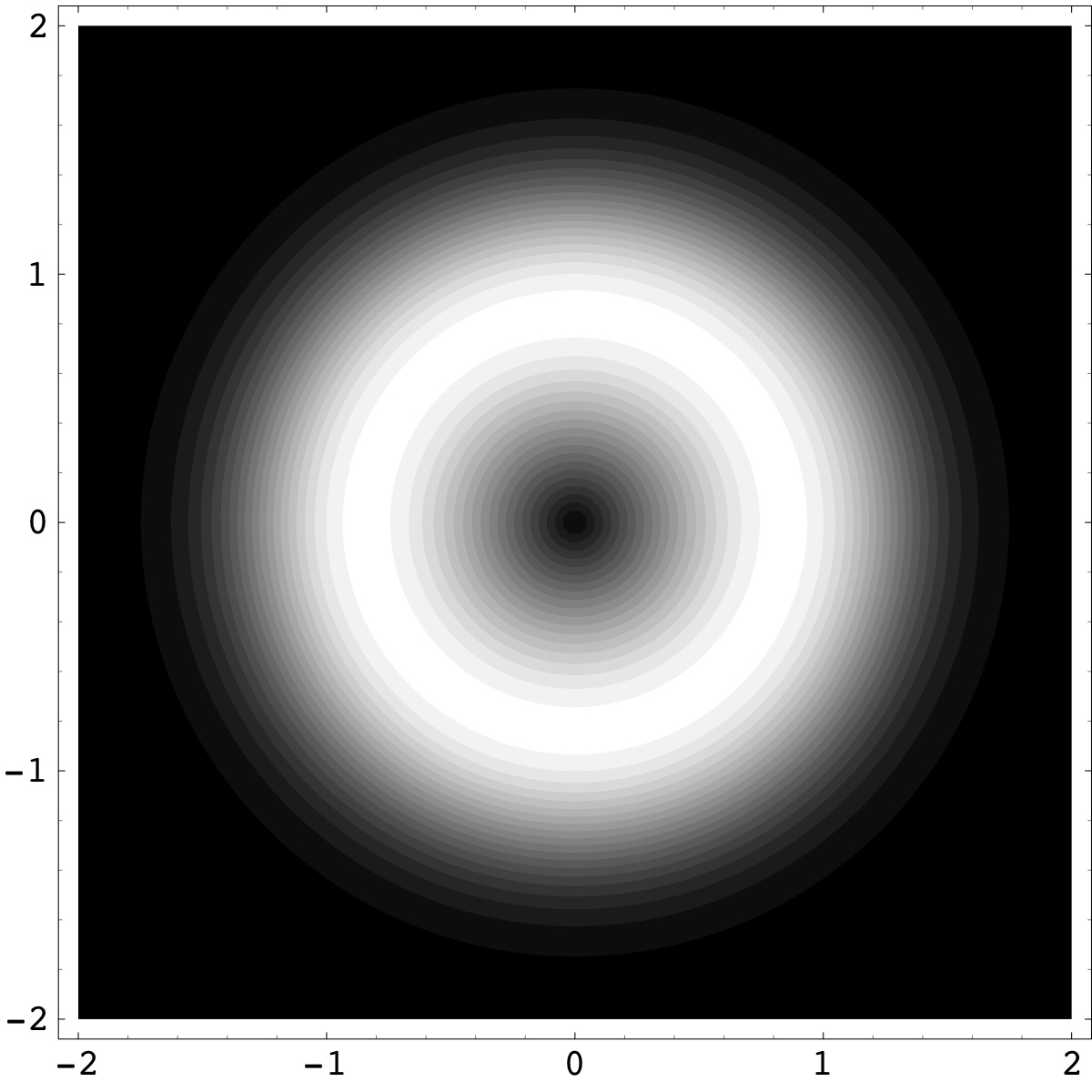
Figure 4.9. The new chemical species of Santilli magnecules
requires an atomic technology capable of polarizing the orbitals of
individual atoms (rather than molecules) into a toroidal form (rendering
by A. K. Aringazin).
As we shall see later, the above conditions permitted the identification
of new environmental processes in which pollutants in the exhaust are
decreased by increasing the combustion. In particular, fuels verifying
the above conditions are said to admit a full combustion,
namely, they release no uncombusted component in the exhaust, that is,
no HydroCarbons HC, carbon Monoxide CO and other combustible
contaminants present in fossil fuel exhaust.
By proceeding in his typical analytic way, following the identification
of his research goal, Santilli conducted systematic studies on the
realization of the above conditions. To understand the difficulties
facing the conception and production of a new chemical species, one
should recall that atoms have a spherical distribution of their
orbitals, thus normally exhibiting the sole possibility of a valence
bond. Hence, Santilli had to create a new force field in atoms as
a condition to achieve a new chemical species.
The answer originated from Condition 3 that suggested the use of
magnetic forces since all magnetic effect disappear at a given
temperature (called the Curie Temperature). Therefore, Santilli argued
that, in the event a new bond could be based on magnetic fields, all
Conditions 1, 2, 3 would be verified because: magnetic bonds are known
to be weaker than valence bonds; they are expected to be stable under
ambient conditions; and they are expected to decompose themselves at the
combustion temperature that was assumed as being the Curie temperature
of the new bond.
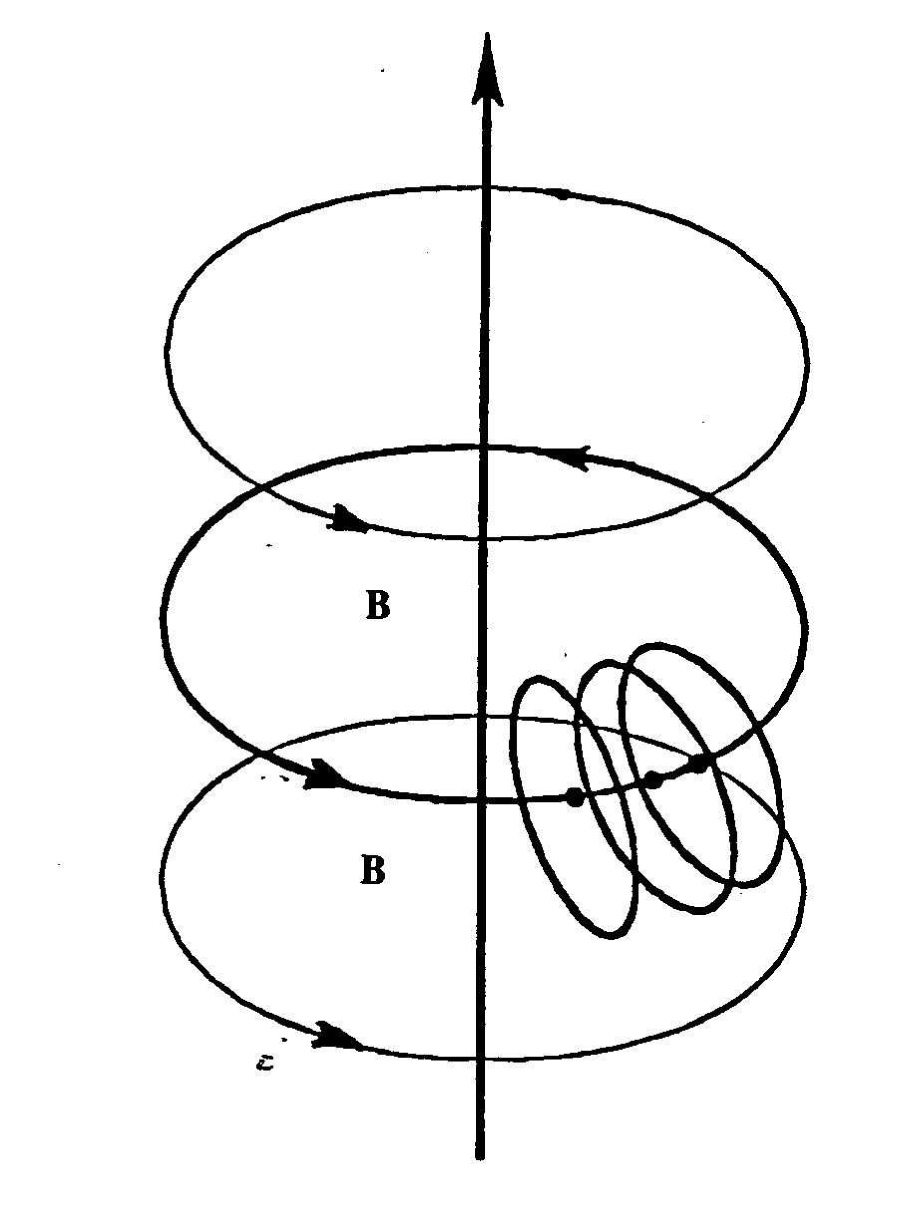
Figure 4.10. The sole known possibility of industrial
production of new fuels with Santilli magnecular structure is given by
submerged electric arcs due to the need for very strong magnetic fields
that are indeed provided by electric arcs at atomic distances. In
particular, the arc performs three important functions: 1) the
polarization of atomic orbitals into toroids with symmetry axis tangent
to the local magnetic force given by a circle perpendicular to the arc
direction; 2) The coupling of different atoms with opposing magnetic
polarities North-South-North-South- etc.; and 3) The compression of
magnetically polarized and coupled atoms toward the arc due to still unknown effects
.
Santilli argued that the possible control of the distribution of atomic
orbitals, from their conventional spherical form to a toroidal
form would indeed create a magnetic dipole North-South along the
symmetry axis, thus creating the needed new field in atoms that normally
have none.
A huge difficulty then emerged because it is known in the technical
literature that the control of the orbitals of atomic electrons requires
extremely strong magnetic fields of the order of 1010 Gauss
or more, namely, magnetic fields at least 1,000 time stronger then the
biggest magnetic field available at the U. S. Large Magnet Laboratory in
Tallahassee, Florida.
By again proceeding in his analytic way, Santilli conducted a systematic
search of engineering means for exposing atomic orbitals to the needed
very strong magnetic fields, and concluded his studies with the
selection of DC electric arcs submerged within a fluid (a gas or
a liquid). In fact, in this case, the magnetic field M follows the known
law
(4.26) M = k A/r,
where A represents the number of Amperes of the arc, r the distance from
the arc and k a constant depending of the selected units whose value is
here irrelevant. Therefore, at atomic distances from the arc, that are
of the order of 10-8 cm, and for currents of the order of
103 A, the magnetic field M is indeed of the desired order
of 1011 Gauss.
In early 1998, Santilli introduced his new chemical species he called
magnecules to distinguish them from conventional
molecules, the former having the new magnecular bond, the
latter being characterized by the conventional valence bond, the
new species being defined as follows:
SANTILLI MAGNECULES: Are given by clusters comprising individual
atoms, such as H, C, O, etc., dimers, such as H-O, C-H, etc., and
ordinary molecules, such as C-O, CO2, etc., bonded together
by opposing magnetic polarities of toroidal polarizations of atomic
orbitals plus opposing nuclear and electron magnetic polarizations.
Electric polarizations are evidently expected to participate in the
magnecular bond, although they are notoriously weaker and much more
unstable than magnetic bonds.
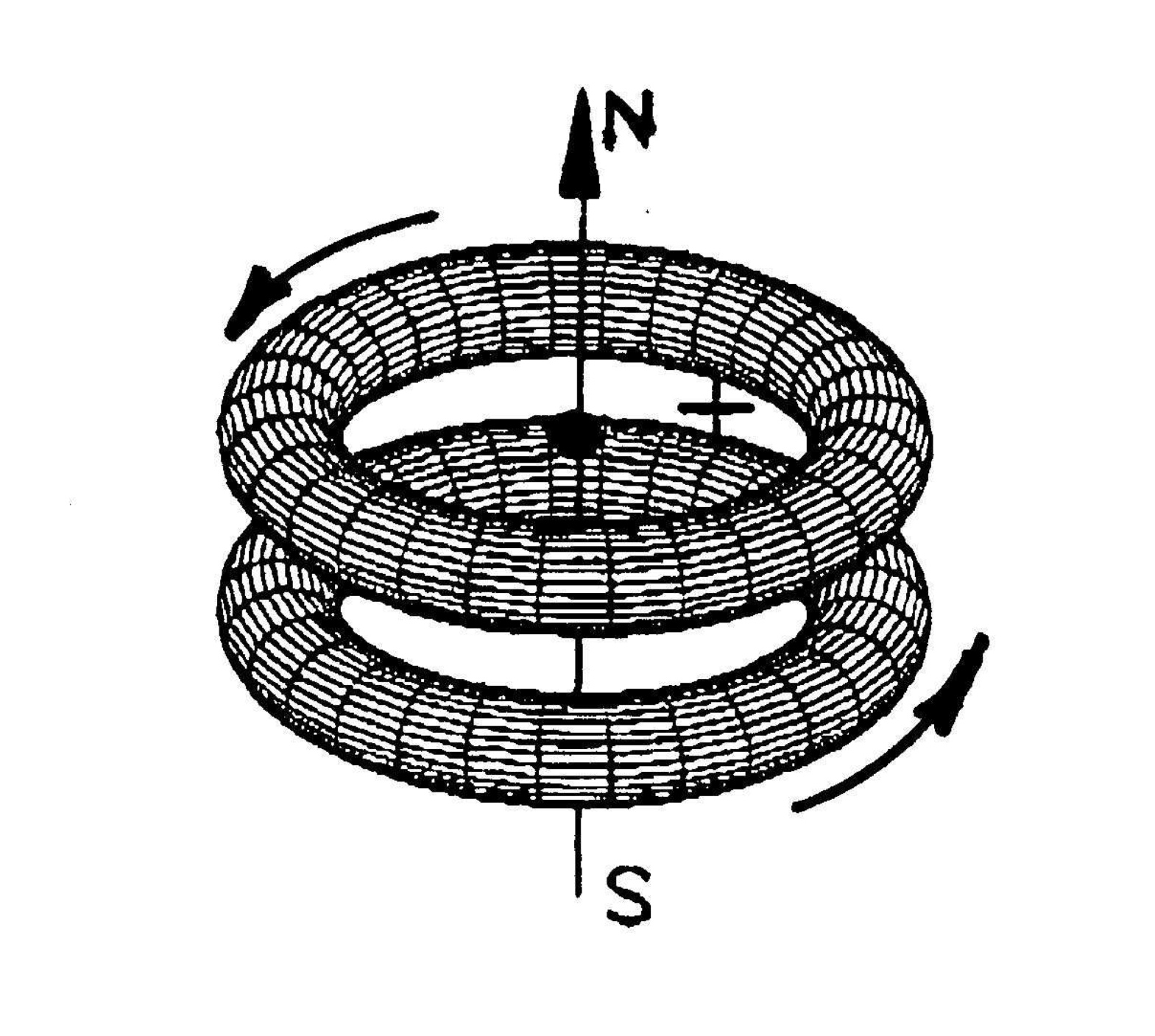
Figure 4.11. A conceptual rendering of an "elementary
magnecule" comprising two identical or different atoms whose bond is
entirely of magnecular character, namely, originating from opposing
polarities North-South-North-South of the toroidal distributions of
orbitals, as well as the polarization of nuclear and electron magnetic
moments.
In the historical paper of 1998 Santilli conducted a quantitative
identification of the magnetic field caused by electrons rotating at a
speed close to the speed of light within a toroidal polarization, and
concluded that such a magnetic field is about 1,315 stronger than the
nuclear magnetic field. This calculation was independently verified for
the first time by M.G. Kucherenko and A.K. Aringazin in the paper
Estimate of the polarized magnetic moment
of the isoelectronium in the hydrogen molecule
M.G. Kucherenko and A.K. Aringazin, Hadronic J. Vol. 21, 895-902 91998.
Additional comprehensive studies were conducted by A. K. Aringazin, as
in the paper
Toroidal configuration of the orbit of the
electron of the hydrogen atom under strong external magnetic
fields
A.K. Aringazin,
to appear in Hadronic J. (2001).
4.3C. Detection of Santilli magnecules
There should be no surprise on the fact that no new chemical species were
discovered from the pioneering studies by Avogadro, Canizzaro and
others of the middle of the 19th century until Santilli studies at the
end of the 20th century. In fact, all available analytic methods and
equipment have been conceived, developed and established for the
detection of molecules, and NOT for magnecules. Any belief that
Santilli's magnecules can be detected or denied via the use of
conventional molecular means constitutes a mere illusion at best, the
results emerging from such biased approaches being called by Santilli
"experimental beliefs."
The serious scientist should remember that, by conception and
construction, magnecules have a bond much weaker than that of
molecules. Consequently, all analytic methods that are indeed very fast
and efficient for the detection of molecules, usually destroy the very
magnecular species to be detected, e.g., because the energy of
ionization beams is much bigger than that for magnecular separation
while being unable to cause any molecular separation. Also, all magnetic
bonds and effects are known to have a Curie temperature at which they
disappear. Therefore, any claim of lack of existence of magnecules via
Gas Chromatographers (GC) and other equipment using thermal and/or high
temperature detection processes, is purely nonscientific, since the
thermal process destroys the very species to be detected.
By far the biggest difficulties were experienced by Santilli in the
experimental verification of his new species, not only because of the
deplorable conditions of analytic chemistry at the end of the 20th
century for which no basic novelty was believed as being possible.
Hence, when faced with anomalous results, rather than admitting possible
novelty, analysts would enter into incredible gyrations and
manipulations of the equipment for the specific intent of eliminating
any possible novelty, and then claiming lack thereof.
A typical example in gas chromatography is the reduction of a peak in
the mass spectrum, let us say, at 250 atomic mass units (amu) to its
components H2, CO, etc., and then claim absence of a new
species, when the detected conventional molecules are the
constituents of the peak at much lower amu, thus resulting in a
pure "experimental belief" without scientific content.
Additional difficulties were created by the widespread practice of
conducting only one detection and then claiming a final
"experimental result," while in reality no result can be claimed to be
scientific on serious grounds without at least a second
verification with a different instrument. This practice is
particularly insidious for magnecules because "experimental beliefs"
obtained with one analytic equipment can be solely dismissed with a
second independent verification.
Following a systematic study of all available equipment, Santilli
selected the use of a Gas Chromatographer Mass Spectrometer equipped
with an InfraRed Detector (GC-MS/IRD) because that instrument allows the
study of the same cluster, firstly, in the mass spectrum and,
secondly, under the IRD. In the event the GC-MS and the IRD are used
separately, the MS peak would not generally appear in the IRD when of
magnecular nature, thus leading to manipulations of experimental data
and illusions of scientific process.
Santilli then conducted a nation wide search for a GC-MS/IRD to discover
that no academic or commercial analytic laboratory in the U.S.A. had
none since GC or GC-MS alone are very effective for molecular
detections, thus leading to the widespread tendency of avoiding any
verification of the results via a different equipment. Continued search
revealed that military and forensic laboratories do generally have
GC-MS/IRD due to stringier requirements for verification and accuracy.
Following such an extensive search, Santilli finally located the
availability at the McClellan Air Force Base near Sacramento,
California, of a GC-MS/IRD consisting of a HP GC model 5890, a HP MS
model 5972, equipped with a HP IRD model 5965. Santilli visited said
basis on June 19, 1998, with samples of gas produced via an arc between
graphite electrodes submerged within distilled water. Following
confirmation of the needed equipment, the GC-MS/IRD had to be used in
way rather unusual for molecular tests, such as:
1) The feeding line had to be the largest available because feeding
lines with small sectional area (of a fraction of 1 mm2),
that work perfectly well for molecular species, do not allow the passage
of magnecular species with large amu due to an anomalous adhesion of
magnecules to the internal walls of the feeding line;
2) The temperature of the column had to be the lowest possible because
high column temperature, while excellent for rapid detection of
molecules, destroy in part or in full the magnecular species to be
detected;
3) The elusion time had to be the longest admitted by the instrument
(and set at 21 minutes) to allow the proper separation of magnecules
into clusters appearing in the mass spectrum, while short elusion times
so effective for the separation of molecules, may provide the
superposition of magnecular clusters without proper separation, thus
with ambiguous results.
Following considerable perplexities due to the unusual character of the
requests, the analysts at the McClellan Air Force Base did implement all
unusual requests by Santilli and, in so doing, produced on June 19, 1998
the first experimental evidence on the existence of the new chemical
species of magnecules reported in the figures below.
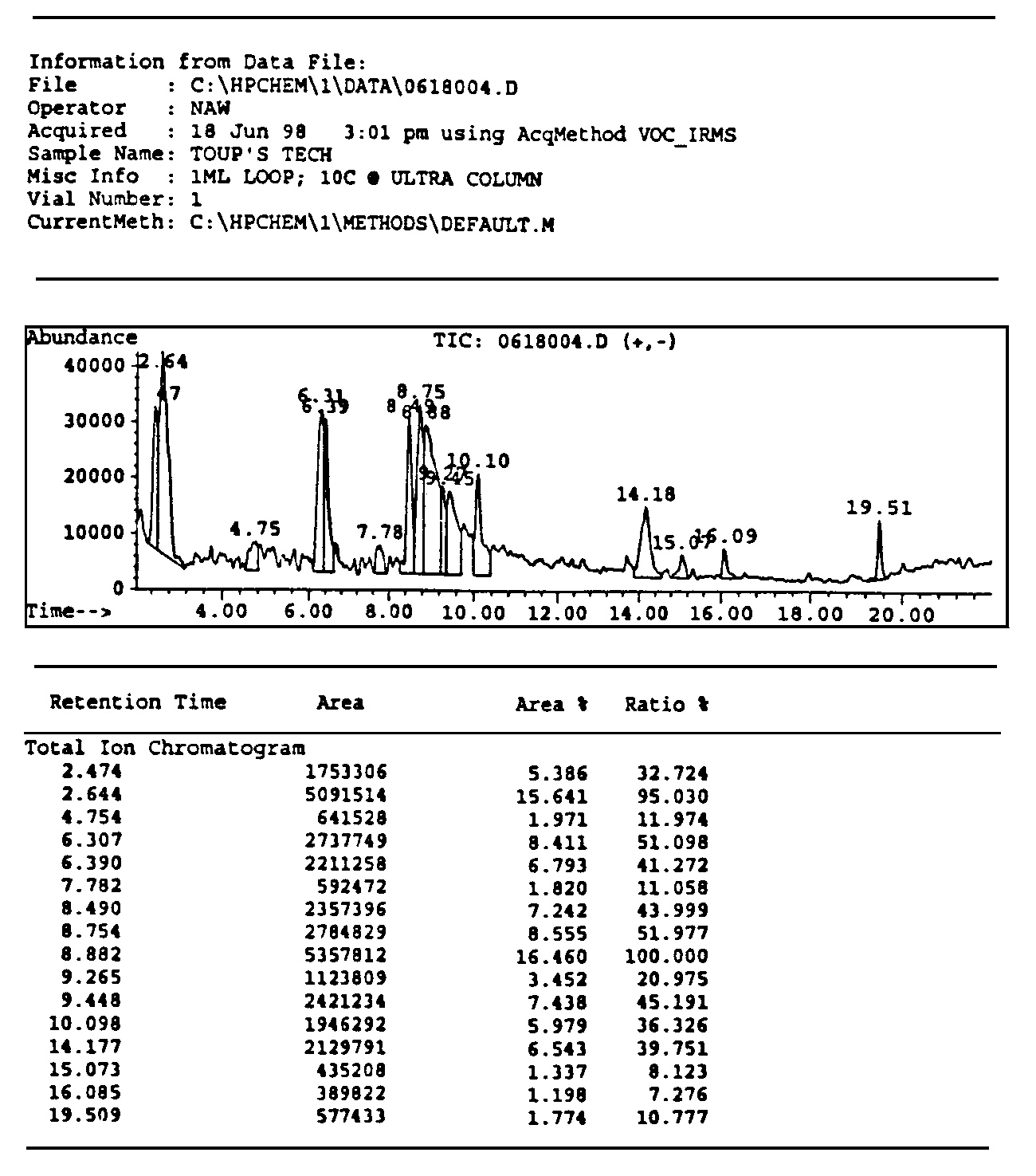
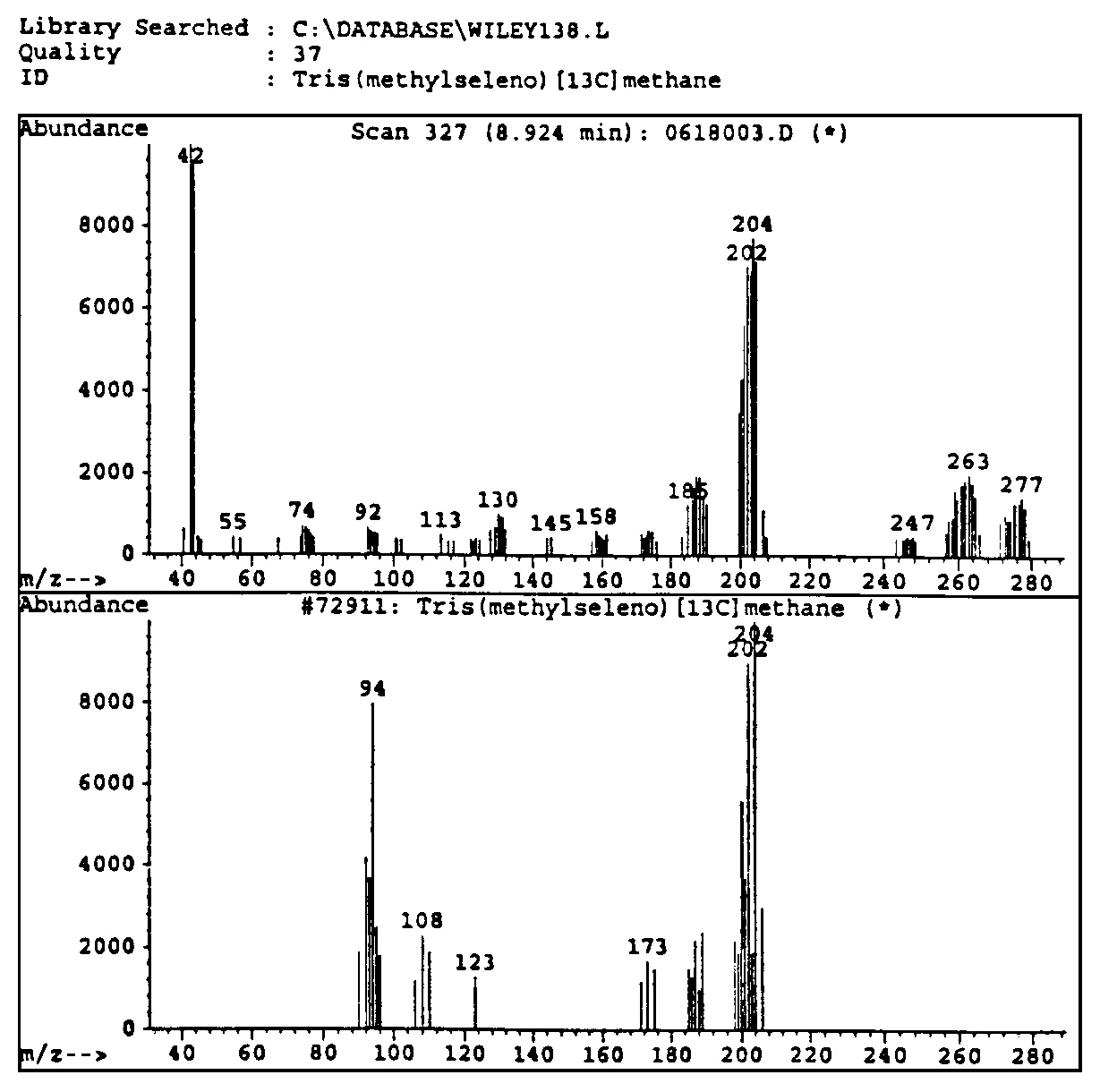
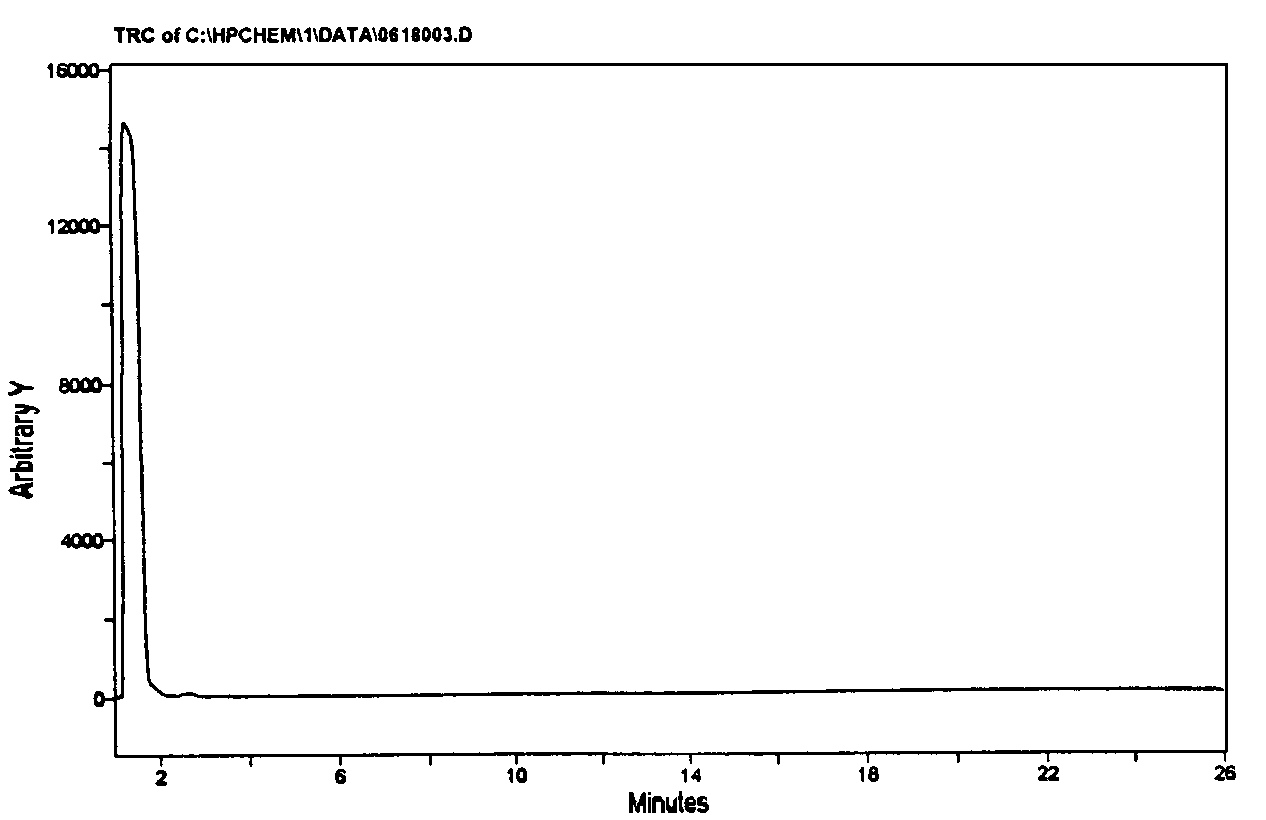
Figure 4.12. The historical printouts on the original
detection of Santilli magnecules achieved on June 19, 1998, at the
analytic laboratories of McClellan Air Force Basis near Sacramento, CA,
via a HP GC model 5890, and a HP MS model 5972 equipped with a HP IRD
model 5965. The test was conducted on a gas with magnecular structure
produced by Santilli via an electric arc between graphite electrodes
submerged within distilled water. According to quantum chemistry, the
heaviest expected species was CO2 at 44 amu. For this reason,
the analysts set the scan between 40 amu and 400 amu, the latter being
the instrument upper limit. At the appearance of the numerous unexpected
species of the top figure, all much heavier than 44 amu and in
macroscopic percentages, the analysts showed some surprise. Hence, they
requested the computers of the Air Force Basis (containing all known
molecules in excess of 500,000 species) to identify the peaks. At the
failure of identifying any of the anomalous peaks (see the example in
the middle), the analysts indicated to Santilli that he had apparently
produced "new molecules" not yet known to them. At that point, Santilli
requested the analysts to inspect the same anomalous peaks under the
IRD. Following due procedures, the scan at the bottom appeared solely
showing the IR signature of CO2. The evidence that none of
the mass peaks of the top scan had any IR signature as in the bottom
scan caused considerable excitement due to clear impossibility for these
large mass peaks to be molecules since only the hydrogen and very few
other light molecules have the perfectly spherical shape necessary to
have no IR signature. The analysts of the McClellan Air Force Basis
expressed their congratulations to Santilli and released a signed
statement of novelty reproduced in the historical 1998 paper as well as
in FHC.
An additional experimental evidence is given by the anomalous
adhesion of gases with a magnecular structure, namely, their
adhesion to solid or liquid substances irrespective of whether
paramagnetic or diamagnetic. This anomalous characteristics is
established by the blanks of GC-MS that, following the flushing of the
magnecular gas with an inert gas, show essentially the same peaks as
those of the scan with the gas, to such an extent to require flushing
with an inert gas at high temperature to recover conventional blanks,
namely, those with no appreciable peaks except for background (see
Figure 4.21 below). This feature evidently confirms the magnetic
polarization of the gases here considered as well as its existence at
the level of individual atoms, a condition necessary for adhesion via
polarization by induction to both paramagnetic and diamagnetic
substances. The feature also has a number of important industrial
applications, such as the elimination of the seepage through containers
walls for hydrogen when treated to have a magnecular structure (see
Section 4.4E).
For additional experimental verification, we refer the serious scholar
to the literature in the field, such as:
"Foundations of Hadronic Chemistry,
with Applications to New Clean Energies and Fuels"
R. M. Santilli,
Kluwer Academic Publishers (2001)
Hadronic
Mathematics, Mechanics and Chemistry, Volume V:
R. M. Santilli, International Academic Press (2008)
The novel magnecular species of hydrogen and oxygen
with increased specific weight and energy content
R. M. Santilli,
Intern. J. Hydrogen Energy Vol. 28, 177-196 (2003)
Figures 28-29 of the latter references are significant because they
present the scan of a GC-MS/IRD similar to that at the McClellan Air
Force Bases, operated by a forensic laboratory in Largo, Florida with
similar results (unknown heavy peaks in the MS without IR signature),
although with the accumulation of the MS peaks due to the short elusion
time.
4.3D. Magnecular structure of H3 and O3
(1998)
As it is well known, GC-MS routinely detect the species H3 at
3 amu and O3 at 48 amu. Their interpretation in quantum
chemistry is that via a conventional valence bond.
Santilli never accepted such an interpretation for various reasons. To
begin, the deep correlation-bond of valence electrons is in single
couplings as in Figure 4.2, thus creating a boson with total spin
0. Consequently, Santilli argues that it is impossible for quantum
mechanics and chemistry that a fermion with spin 1/2, the electron, can
bond to a boson with spin 0, the isoelectronium valence pair.
As recalled in Section 4.1, the notion of valence in quantum chemistry
is not quantitative for various insufficiencies, including the absence
of the correlation-bond of the valence electrons, specifically, to
electron pairs. It is then evident that, in the absence of such a
restriction, the species H3 and O3 can have a
valence bond. However, such an interpretation is faced with serious
inconsistencies, such as the prediction that all substances are
paramagnetic (Section 4.2C).
Additionally, nature establishes beyond doubt that the valence bond is
for electron pairs. Hence, the valence bond for the species
H3 and O3 requires that only two out of three
atoms are bonded at any given time. Santilli has proved that such an
interpretation is disproved by the binding energies of H3 and
O3, namely, the predicted binding energy is in dramatic
disagreement with experimental values.
In view of the above and other evidence, Santilli proposed in FHC that
the species H3 and O3 have a magnecular
structure of the type
(4.27) H3 = (H - H) x H, O3 = (O - O)
x O,
namely, they comprise ordinary molecules H2 and O2
with valence bond plus a third atom with magnecular bond (see Figure
4.13), thus being magnecules according to their definition. In any case,
it is known that ozone O3 is formed under electric
discharges precisely as needed for the production of magnecules
(Section 4.3B), and a similar mechanism holds for the H3.
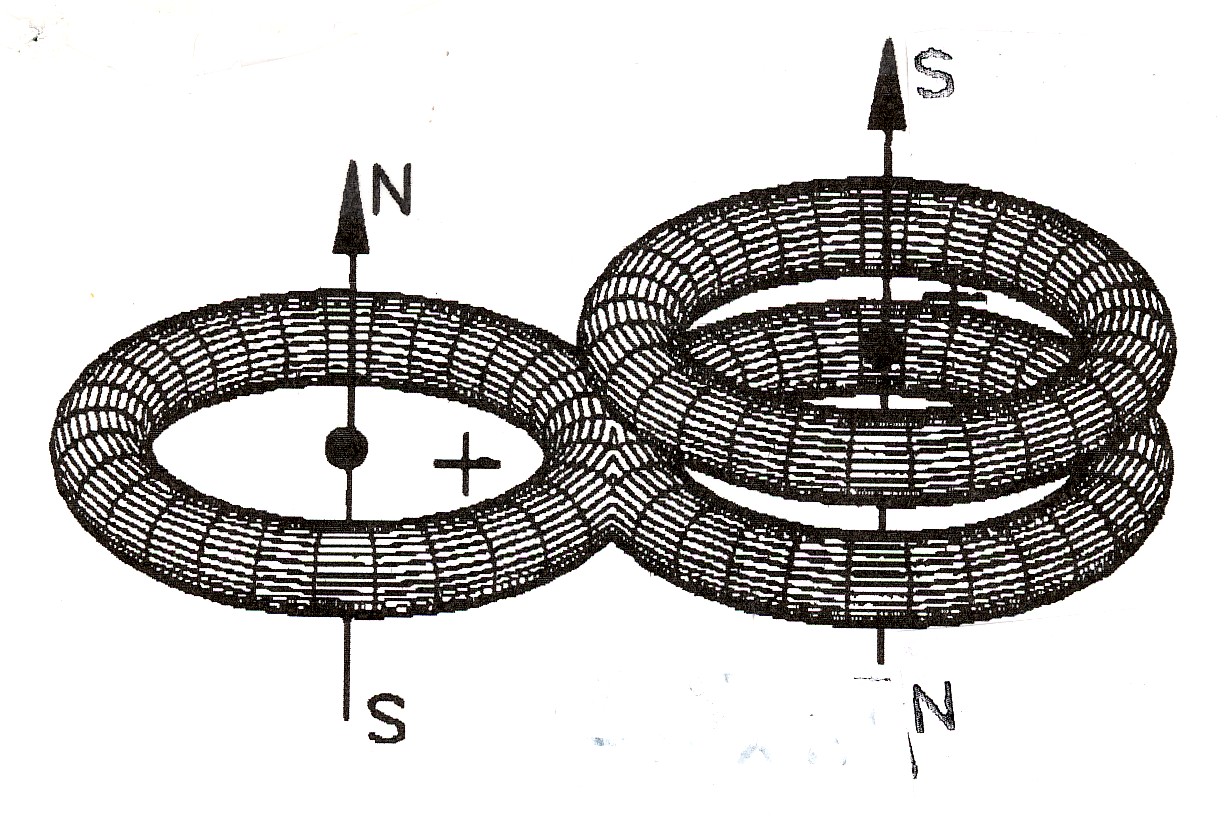
Figure 4.13. Quantum chemistry suggests that the valence, in
one of its various nomenclatures without quantitative treatments, is
responsible for all possible species existing in the universe,
expectedly, until the end of time. Consequently, for quantum chemistry
the species H3 and O3 are characterized by valence
bonds. Santilli has identified numerous inconsistencies of such an
interpretation and shown that said species have instead a magnecular
structure comprising conventional molecules H2 and
O2 with a third atom under magnecular bond as shown in the
figure. In fact, ozone O3 is formed under intense electric
discharges that separate O2 molecule as a condition to have a
free oxygen atom, jointly polarize the O2 molecule, and
align them as in Figure 4.10, thus providing a natural representation of
its formation, let alone of its structure in a way compatible with
experimental data. The same holds for
H3.
4.3E. Magnecular structure of liquids and solids (1998)
Another notion of quantum chemistry rejected by Santilli since his
graduate studies is the interpretation of the water liquid state as
being due to "H-bridges" or similar conjectures, because, as it is the
case for the valence, the notion is a pure nomenclature without the
explicit identification of a force binding the water molecules together,
without the proof that such a force is indeed attractive, and without
showing that such an attractive force represents experimental data.
In his historical monograph FHC, Santilli proposed that the liquid
state of water as well as of other liquids has a magnecular
structure, namely, the bond between the water molecules is of
dominant magnetic character, evidently with an inevitable component
originating from electric polarizations. The view was based on various
reasons, such as:
1) An inspection of the water molecule in its natural state as depicted
in Figure 4.7, reveals that the orbitals of the H atoms do not have a
spherical distribution, but instead have a toroidal one, thus possessing
a natural magnetic field North-South along the symmetry axis. The same
must occur for the orbitals of the corresponding valence electron of the
O atom much along the Santilli-Shillady strong valence bond of Figure
4.5. It is then quite natural to interpret the bond between water
molecules in the liquid state as caused by attractive, opposite,
magnetic polarities North-South-North-South of the toroidal
polarizations of the H and O orbitals, as in Figure 4.14.
2) All available valence electrons in the water molecule are strongly
bonded, as established by the high value of energy needed for molecular
separation. Consequently, any belief that the liquid state might
originate from valence bonds is purely nonscientific. The water molecule
is also neutral and, consequently, the sole use of electric
polarizations as the entire origin of the bond for the water state fails
to provide any quantitative representation of experimental data, such as
the energy needed for the breaking of the liquid state into a gaseous
form. These occurrences leave magnetic polarizations as the sole
possible or otherwise plausible origin of the bond in the liquid state.
3) The breaking of the liquid state at the boiling temperature is a
confirmation of its magnecular character, since all magnetic bonds cease
to exist at a given temperature. As a matter of fact, the boiling
temperature of water, 100o C, is the Curie temperature of the
magnecular structure of liquid water.
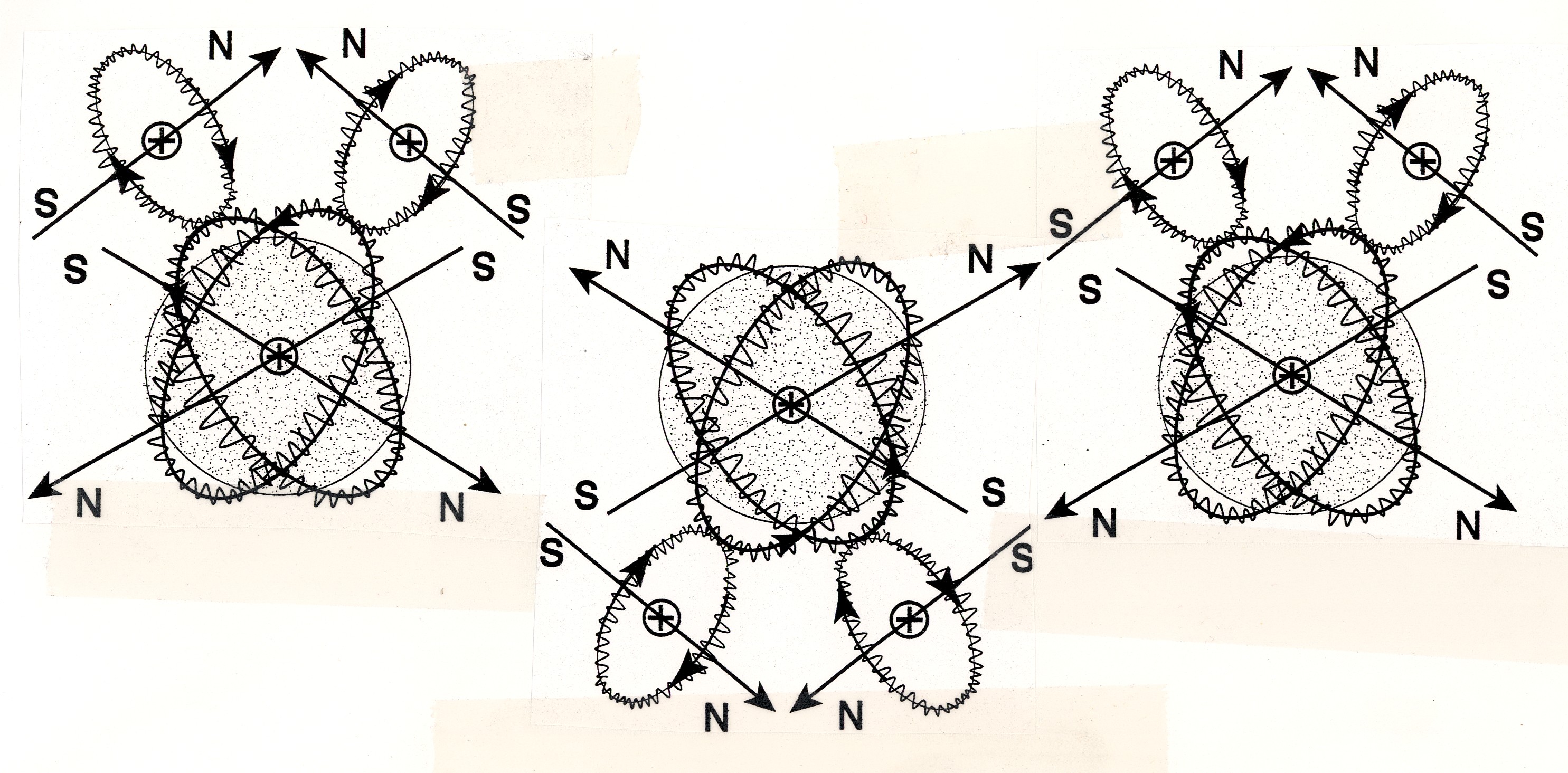
Figure 4.14. A schematic view of the magnecular structure of
the liquid state of water discovered by Santilli and presented in FHC.
The magnecular structure can be confirmed via a mere inspection of the
presence of magnetic fields in the natural state of the water molecule
as in Figure 4.7, whose coupling via opposing polarities
North-South-North-South results precisely in the magnecular structure of
this figure.
In FHC Santilli provides experimental evidence obtained via Liquid
Chromatographers Mass Spectrometers equipped with Ultra Violet Detectors
(LC-MS/UVD, the equivalent for liquids of the GC-MS/IRD for gases) of
the formation of various liquid magnecules via the use of
magnetic fields, including a bond between oil and water. We regret to be
unable to reproduce here this vast experimental evidence.
In FHC Santilli also presents evidence of solid magnecules, such
as fullerines, consisting of hard carbon composites that sublimate at
a sufficient high temperature, thus confirming the magnecular (rather
than the valence) character of the bond. Additional examples of solid
magnecules are given by certain calcium composites initiating with
accretion, then bonding under pressure via magnetic induction, and
finally resulting in the formation of a solid. Additional examples of
gaseous liquid and solid magnecules are nowadays identified rather
frequently.
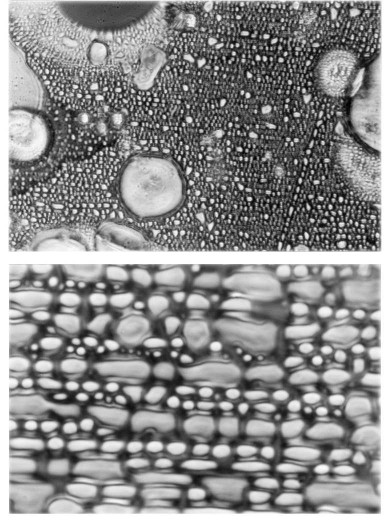
Figure 4.15. The top picture depicts under a 10X magnification
a liquid magnecule composed of a bond between fragrance oil and water,
the lower picture showing its 100X enlarged view. The pictures were
obtained by the Givaudain-Roure Research Laboratories in Dubendorf,
Switzerland. It should be indicated that the bubbles are partially
fragrance oil and partially water, while the magnecule is the entire
dark area of the pictures, which area was transparent water prior to
Santilli's formation of the magnecular bond. Therefore, liquid
magnecules may have specific weights of 10,000 amu or more, and are
generally visible to the naked eye. It is instructive to know that
tests at the same laboratory via an LC-MS conventionally set to identify
oil fragrance molecules, showed no presence at all of any magnecular
species because the analysts refused to use the largest available
feeding line. In so doing, the analysts prohibited the admission in the
LC-MS of the very species to be detected. This occurrence, rather
frequent for analysts whose mind remains set at molecular detections, is
confirmed by the fact that the LC-MS did detect the fragrance oil and
water, but failed to provide any detection at all of the dark liquid of
the pictures.
In Table 8.31, page 371 of FHC, Santilli presents experimental
measurements conducted by the SGS Testing Laboratory of Fairfield, NJ,
according to which liquids and solids exposed to intense magnetic field
exhibit an increase of their density. This important feature is
currently under industrial development for the production, e.g., of
carbon composites with increased specific weight that are of great
importance for automotive and other applications.
4.4 INDUSTRIAL REALIZATION OF FUELS WITH MAGNECULAR STRUCTURE
4.4A. Catastrophic forecasts facing mankind
As it is well known, mankind is nowadays extremely dependent on
petroleum fuels, with about one billion cars, five millions trucks, five
hundred thousand planes in daily use, plus an unknown number of
military, industrial and agricultural vehicles, with clear trends for
rapid increase of fuel consumption.
As it is equally well known, world petroleum reserves and production
either have already reached their peak, or their maximal capacity is
only a question of a few years, with catastrophic risks for mankind due
to the expected exponentially increasing costs under shortages of
petroleum fuels, to such a level to cause disruptions of the very
fabric of our societies, all the way to nuclear wars.
Only totally irresponsible people can sit and wait for these disasters
to occur and do nothing, since NOW is the time to initiate corrective
measures. Along these lines, the only possible, or otherwise credible
way to prevent said catastrophes is to develop an alternative fuel that,
as Santilli puts it, to be really effective, must verify the following::
CONDITION I: The alternative fuel must be suitable for use in
existing engines without structural modifications, as a prerequisite to
really have a serious control of our future. For instance, fuel cells
operated car, while fully commendable, cannot provide a real solution
because they cannot replace one billion existing cars prior to the
indicated catastrophes. Similarly, hydrogen as a fuel for internal
combustion engines, even though equally warranted for development, is
not a solution because its use requires a structural change of existing
engines, and similar occurrences hold for other alternative fuels.
CONDITION II: The alternative fuel must be produced from a widely
available feedstock other than petroleum or food. This condition
eliminates ethanol produced from corn and similar alternative fuels as
viable alternatives. In any case, a large scale production of a fuel
from food would cause per se catastrophic problems in food shortage,
price, etc.
CONDITION III: The production of the alternative fuel must be energy
efficient, in the sense that the energy content of the fuel must be
bigger than the energy used for its production. This condition
eliminates ethanol., hydrogen and other alternative fuels under their
current production methods since their energy content is a fraction of
the energy used for their production.
CONDITION IV: The alternative fuel must be cost effective as a necessary
prerequisite for its actual use by individuals with consequential
prevention of the indicated catastrophes. This condition eliminates
ethanol, hydrogen and other fuels as a viable alternatives because they
are currently more expensive than gasoline for the same energy content
and are predicted to remain so under known production technologies..
CONDITION V: The alternative fuel must be environmentally acceptable on
global grounds, including production, storage and combustion. This
condition eliminates hydrogen as currently produced from fossil fuels
or via the electrolytic separation of water under commercially available
electricity because in both case the pollution caused by hydrogen
production is bigger than that caused by gasoline production and
combustion. Additionally, current methods for hydrogen production and
combustion cause "oxygen depletion," one of the most serious
environmental problems I introduced at the 2000 World Hydrogen
Conference in Munich, Germany, consisting of the permanent removal of
breathable oxygen in our atmosphere and its conversion into forms not
usable for breathing. In fact, fossil fuel combustion converts
O2 into CO2 that is no longer recycled by our
plants due to the enormous volumes of daily production. Hydrogen is a
beautiful fuel when is produced from:
1) Renewable energy sources, such as solar, wind or hydro-energy;
2) Via the electrolytic separation of water; under the additional
condition that
3) The produced oxygen is freely released in the atmosphere for being
recaptured by hydrogen combustion as a necessary condition to maintain
the current oxygen percentage in our atmosphere.
By contrast, the oxygen produced in electrolytic separation of water is
nowadays sold and used for fertilizers, food, metal cutting, and other
uses none of which make oxygen available for hydrogen combustion. Under
these conditions, hydrogen combustion causes a very serious oxygen
depletion evidently given by the conversion of atmospheric oxygen into
H2O, that is not appreciably recycled by plants into
breathable oxygen also in view of the very large volume of water
existing in our planet.
To understand the gravity of oxygen depletion, responsible
individuals should note that we have accumulated in our atmosphere an
estimated number of one billion tons of excess CO2 from
fossil fuel combustion and hydrogen production. The O2 in
CO2 was originally breathable oxygen and it is now no more.
But O2 constitutes about 72 % of CO2. Therefore,
we have eliminated from our planet an estimated number of 720 million
tons of breathable oxygen.
In the event hydrogen is allowed to have a large scale automotive
and other uses, because of the depletion of oxygen into CO2 caused by
its current production plus the depletion of oxygen into H2O
caused by its combustion, human life on Earth would be extinct in a few
years because of the reduction of breathable oxygen below the level
needed to sustain human life.
The understanding of Santilli's discoveries by responsible individuals
requires the knowledge that his fuels with magnecular structure,
generically called magnegases, are the only known fuels meeting
Conditions I-V while being industrially available NOW. Additional
understanding of the societal relevance of Santilli's discoveries will
be presented in the next chapters in relation to truly new and clean
energies.
4.4B. Santilli hadronic reactors (1998)
Submerged electric arcs were discovered in the 19th century for the
repair of metal ships. The production of a gas and its combustible
character were discovered at the same time by sailors who used to lit up
the gas emerging from underwater welding and called it "fire on water."
The clean burning character of the gas was also known at that time.
Despite the above promising features, submerged electric arcs did not
reach industrial relevance for the production of a combustible gas
since their discovery in the the 19th century until Santilli's studies
in the late 1990s.
Recall from Section 4.3 that Santilli had selected submerged electric
arcs for the production of his new magnecular species. Therefore,. he
initiated the systematic study of submerged electric arcs in 1998. By
proceeding with his typical analytic method, Santilli first identified
the main limitations of submerged electric arcs, and then conducted
systematic theoretical and engineering studies for their resolution by
using his knowledge of hadronic mechanics and chemistry.
In essence, electric arcs submerged within a stationary liquids have a
very low Efficiency E defined as the ratio between the volume V
in scf of gas produced and the electric energy Eelec needed
for its production expressed in Wh
(4.28 ) E = V / Eelec.
Elctric arcs between carbon electrodes are indeed very efficient in the
separation of water molecules by producing a plasma of mostly ionized H,
O and C atoms around the electrode tips. However, the passage of the
arc through the H and O atoms causes their combustion into
H2O via an implosion, thus resulting in a very low efficiency
E, with consequential excessive cost for the combustible gas, because
most of the separated water molecules are then recombined.
Stationary submerged electric arcs have additional shortcomings, such
as the production in the plasma of an excessive percentage of
CO2, measured up to 18 % prior to combustion (and 27 % in the
combustion exhaust), due to the synthesis of CO in the presence of O
atoms while being traversed by the arc.
Santilli conducted systematic engineering studies for the resolution of
the above insufficiencies by developing a new method called
PlasmaArcFlow (PAF, patented and international patents pending),
consisting in continuously flowing the liquid feedstock through the arc,
then controlling the cooling down of the plasma in the surrounding
liquid, and controlling the formation of the combustible gas with
magnecular structure, while the latteris cleaned by its bubbling through
the liquid. This new PAF process does indeed allow the production
of a clean burning, cost competitive magnecular gas ready for combustion
at the time of its production without any need for additional refining.
PlasmaArcFlow Refineries are known scientifically as Santilli
hadronic reactors because of their conception and engineering
realization via the use of hadronic mechanics and chemistry due to
excessive divergences between the predictions of quantum theories and
experimental data outlined in Figure 4.16 and Chapter 6. In this
respect, Santilli states:
As an editor of various journals, I continue to receive papers to
this day attempting to represent the structure of the hydrogen atom with
Newtonian mechanics despite the availability for about one century of
its exact quantum solution. Therefore, it is very easy to predict that
the manifestly nonlinear, irreversible and non-Hamiltonian processes
caused by submerged electric arcs will indeed continue to be studied
with the manifestly linear, reversible and Hamiltonian special
relativity and quantum mechanics, despite the availability of
numerically exact and invariant solutions via the nonlinear,
irreversible and non-Hamiltonian genomechanics, thus resulting in the
mere illusion of serious research.
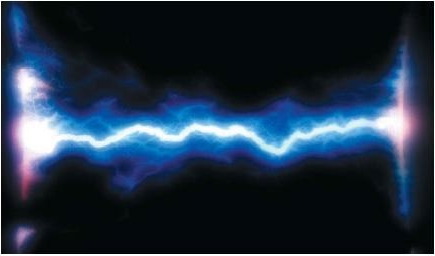
Figure 4.16. A beautiful picture of a DC electric arc between
graphite electrodes submerged in distilled water that constitutes,
jointly with the valence and other events, a case of clear
inapplicability of 20th century theories. To begin, Maxwell's equations
and special relativity are certainly applicable to an arc in vacuum (or
at best in air), but they are fundamentally inapplicable for the same
arc when submerged in water because of the impossibility of
representating: the dielectric character of water; the existence of the
longitudinal, attractive, Ampere force between the two electrodes (that
is basically incompatible with Maxwell's equations); the collapse of the
resistance under a closed arc; and other aspects. Similarly, quantum
chemistry predicts that the gas produced under the considered conditions
is composed of close to 50% hydrogen and 50% carbon monoxide plus traces
of carbon dioxide and other substances, a prediction that is
dramatically disproved by GC-MS analyses (see Figure 4.12).
Additionally, quantum, chemistry predicts the absence of oxygen in the
exhaust, while experimental measurements show the presence in the
exhaust of up to 14 % breathable oxygen, plus having additional
deviations from quantum predictions with errors of the order of at least
ten time the experimental data (see FHC for details). Hadronic
mechanics and chemistry have resolved these insufficiencies for which
reason the equipment producing magnecular gases via submerged electric
arcs are called "Santilli hadronic reactors."
A main feature of Santilli hadronic reactors is their high efficiency
with an energy output that is a multiple of the used electric energy.
This important feature is due to the fact that the primary source of
energy of hadronic reactors is not the electric energy of the arc, but
carbon combustion in the plasma. As a matter of fact, Santilli
conceived his reactors for the primary purpose of developing a new,
cleaner and more efficient carbon combustion.
In the plasma of an electric arc between carbon electrodes submerged
in water we have all chemical reactions of the conventional combustion
of carbon in air, such as the synthesis of CO with the release of 288
Kcal/mole, the synthesis of CO2 with the release of 87
Kcal/mole, and others. Additionally, in the plasma of hadronic reactors
we have the synthesis of H2 with the release of 110 Kcal/mole
that does not occur in conventional carbon combustion in air, thus
showing that Santilli's combustion of carbon in the plasma of an arc has
a bigger energy output of the combustion of the same carbon in air. The
environmental qualities of a carbon combustion via a submerged arc is
evident because all solids and liquid contaminants are trapped in the
liquid and then eliminated by the arc.
To study these aspects, Santilli has introduced first the Scientific
Efficiency (SE) of hadronic reactors given by the total energy
output (as the sum of the energy in the gas Egas plus the
heat acquired by the liquid Eliquid) divided by the total
energy input (as the sum of the used electric energy
Eelectr and the energy of carbon combustion
Ecarb), which Scientific Efficiency is always smaller than
1, and we write
(4.29) SE = (Egas + Eliquid) /
(Eelectr + Ecarb) smaller than 1.
However, hadronic reactors recycle liquid wastes that are generally
very rich in carbon, such as engine oil waste, cooking oil waste, etc.
In this case, the carbon brings an income, rather than carrying a
cost. As a result, Santilli introduced the Commercial Efficiency
(CE) given by the Scientific Efficiency without the carbon energy in the
denominator. Since the latter is a large multiple of the electric energy
(see FHC for details), it is then evident that the Commercial Efficiency
is much bigger than 1, and we write
(4.30) CE = (Egas + Eliquid) /
Eelectr bigger than 1.
In fact, small hadronic reactors (say with 50 kW) have a Commercial
Efficiency of the order of 5, namely, for each unit of electric
energy input, the reactors produce up to five units of energy output as
a combination of thermal energy in the combustible fuel and heat
acquired by the liquid feedstock.
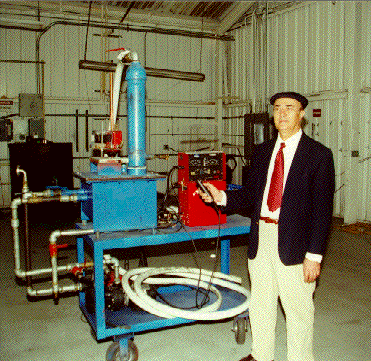
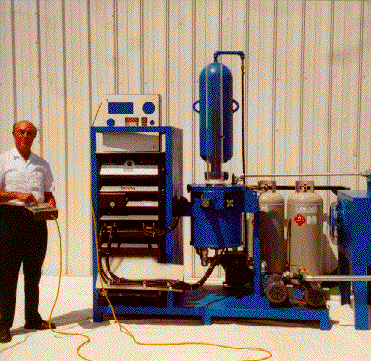
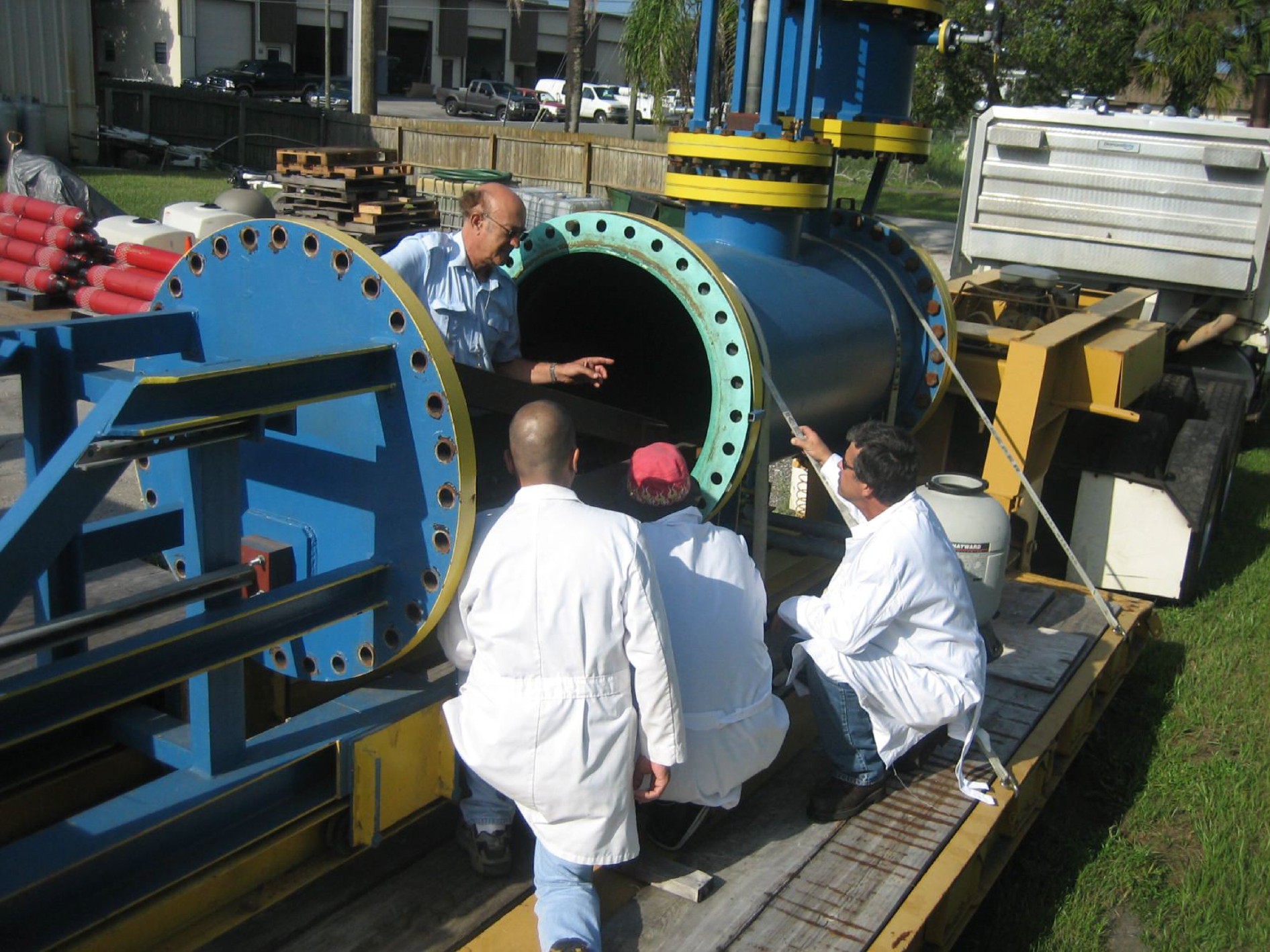
Figure 4.17. Views of some of the various hadronic reactors
built by Santilli for pure research purposes. The top view shows the
first manually operated hadronic reactor built in late 1998 to test the
PlasmaArcFlow principle with minimal costs. Because of encouraging
results, Santilli built a series of sequentially improved research
reactors to test the various aspects of the new technology, the middle
picture showing a completely automatic 50 Kw hadronic reactor built in
2001. Numerous additional research reactors followed. The bottom
pictures shows a large 500 Kw research reactor built in 2005 and still
used by Santilli for various tests, particularly those of nuclear
character outlined in Chapter 6 (the bottom pictures shows from the
left Santilli and three of his technicians: Michael Rodrigujez, John T.
Judy, and Eugene West).
As we shall see in Chapter 6, the above high efficiency cannot be
numerically explained via the sole use of conventional chemical
reactions, such as the synthesis of CO, CO2, etc., and
requires the admission of novel nuclear processes, that, if
properly enhanced, can give an energy output 50 times ot more the used
electric energy.
In regard to cost of the magnegas fuel produced with hadronic
reactors, we recall that, in their industrial version with a minimum of
300 Kw power, operating in the "total mode" at a minimum pressure of
100 psi, at the temperature of 200o F while processing an
oil-base liquid feedstock, hadronic reactors require approximately 70 W
= 220 BTU of electricity for the production of 1 scf of fuel with about
900 BTU/scf, plus 300 BTU of heat acquired by the liquid feedstock with
a total CE = 5.95, the excess energy originating from the chemical and
nuclear reactions in the plasma of the hadronic reactor.
One gasoline gallon contains about 110,000 BTU. Therefore, the
Gasoline Gallon Equivalent (GGE) of magnegas is given by
110,000/900 = 122 scf. The production of one GGE of magnegas requires
122 x 70 W = 8.5 kWh that, at the current rate of $0.08/kWh yields the
electricity cost of $0.68/GGE.
The computation of the total direct cost of one GGE of Magnegas requires
the addition of 30% for other direct costs, including carbon
electrodes, service and amortment of purchase prize over 15 years,
yielding the total direct cost of $1.08 per GGE, less the income
for the recycling of the liquid waste that generally reduces
considerably the direct costs and, in certain special case, can be
bigger than the direct cost.
4.4C. Industrial realization of MagneGas fuel (1998)
Following the above basic research, Santilli conducted systematic
engineering work for the optimization of the PAF method and
industrialization of the process into completely automatic and remote
controlled PlasmaArcFlow Refineries of various dimensions and
powers for the gasification of a variety of liquid wastes into
MagneGas fuel of which he introduced the name as well as the
chemical symbol MG.
In so doing, Santilli obtained the U.S. Patents 6,926,872, 6,673,322,
6,663,752, 6,540,966, and 6,183,604 whose study is suggested for any in
depth knowledge of this new technology. A U. S. corporation was set up
with public listings of its stock that is now in production and sale of
MagneGas in the American continent,
Magnegas Corporation
with affiliated companies for the development of the Magnegas
Technology in India, Europe, Australia and other countries.
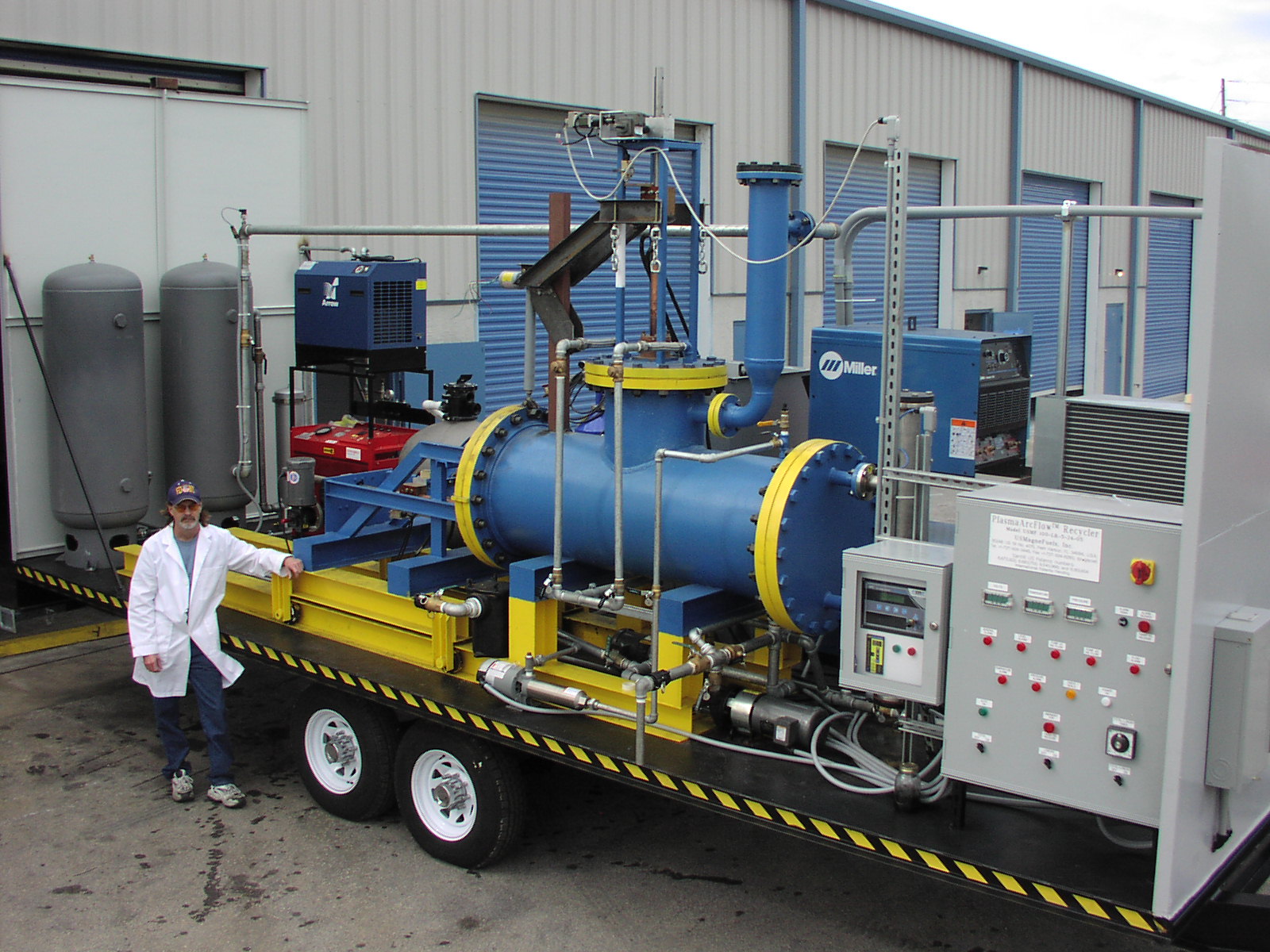
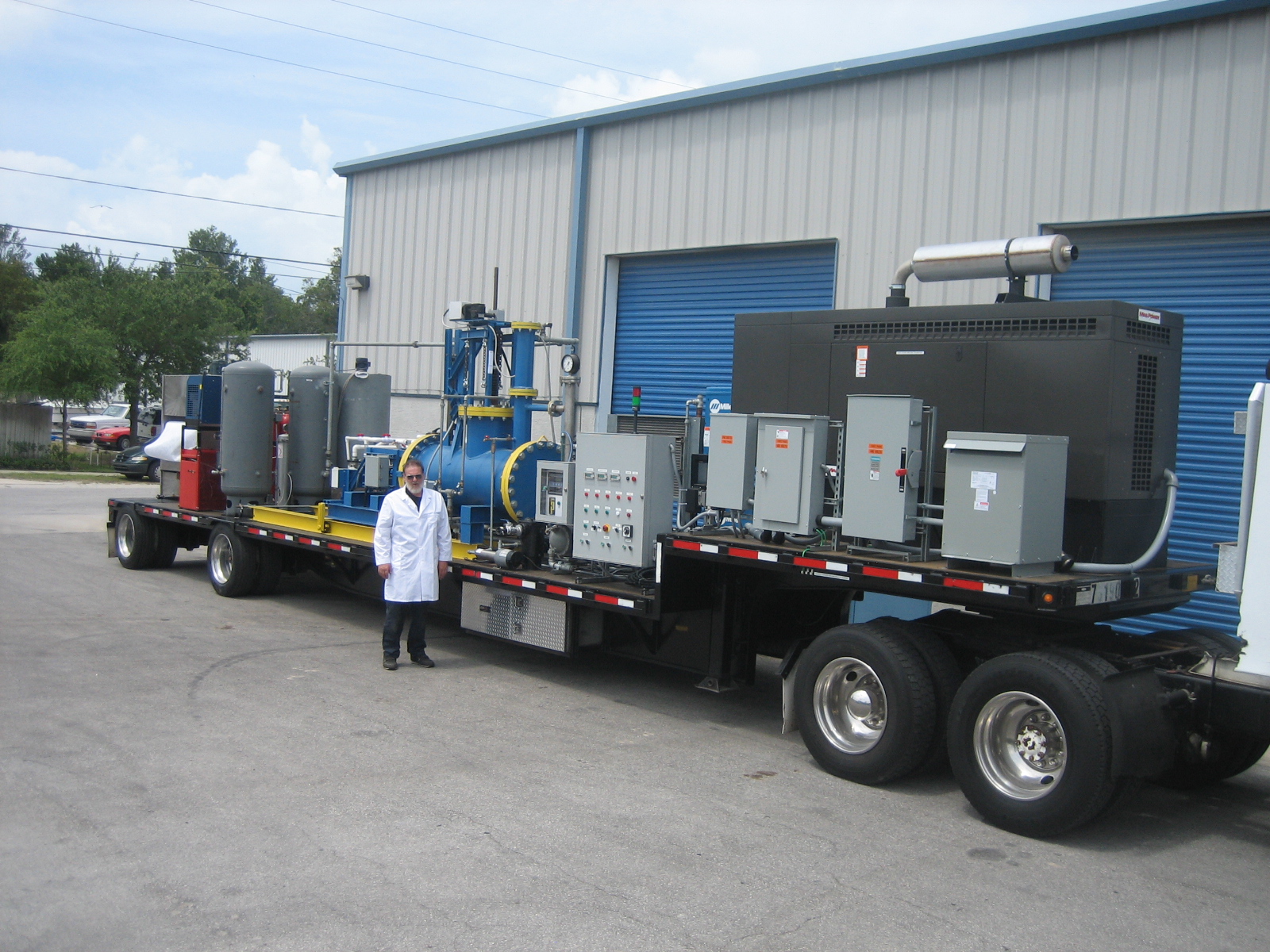
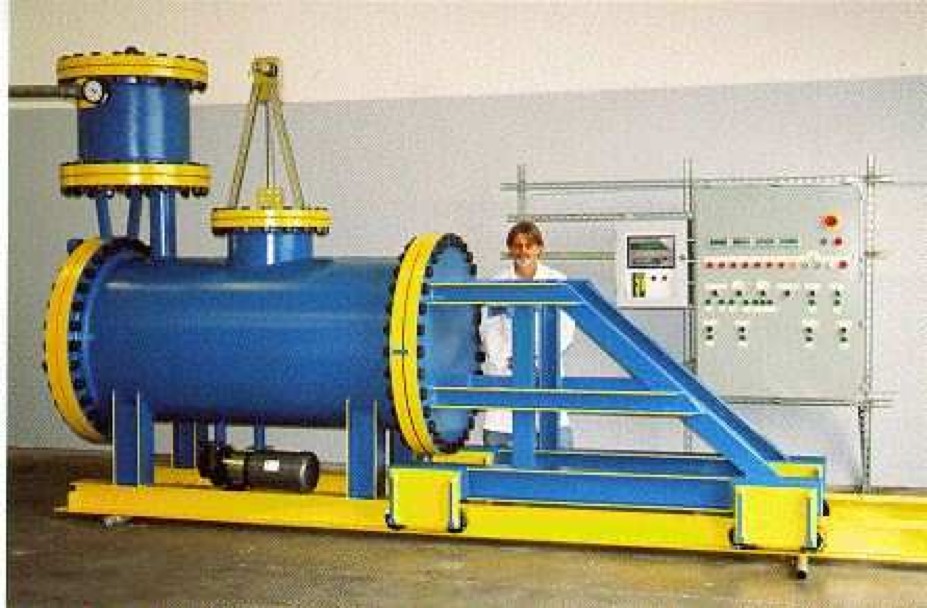
Figure 4.18. A view of various industrial hadronic reactors
built by Santilli: the top view shows a 50 Kw MagneGas Refinery on a
trailer operated by Richard Lyons, one of Santilli's technicians; the
middle view shows a 100 Kw refinery on a trailer operated by John T.
Judy; and the bottom view shows the PlasmarcFlow Module of a 500 Kw
floor mounted industrial Magnegas Refinery.
MagneGas does indeed verify Conditions I-VI of Section 4.4A for an
effective progressive replacement of petroleum fuels in view of the
following main aspects:
1. MagneGas can be effectively used in all existing gasoline
engines (see Picture 4.19 on various cars currently using
magnegas);
2. MagneGas is produced from liquid waste available everywhere in
unlimited volumes, such as engine oil waste, cooking oil waste,
city, farm and factory liquid wastes, etc;
3. MagneGas production is energy efficient, because its energy
content is bigger than the electric energy needed for its
production, as outlined in the preceding sections via the notions of
Scientific and Commercial Efficiencies;
4. MagneGas is less expensive than fossil fuel when produced in
sufficient volume by assuming an income for the recycling of the liquid
waste, as also outlined in the preceding section;
5. Magnegas is environmentally acceptable because it admits complete
combustion, thus having no HC, CO or other contaminants in its
exhaust (see Figiure 4.20).
Additionally, one should bear in mind that MagneGas is industrially
available now. In fact, it is in regular production and sale in
various countries. For additional information, one may visit the
following links:
Fox News video on the Magnegas Technology
Video by Dunedin Water Treatment Plant
Invited
presentation at the United Nations
Video by Magnegas
Corporation
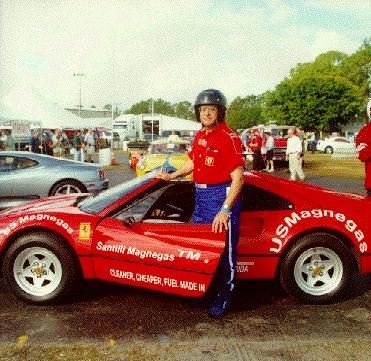
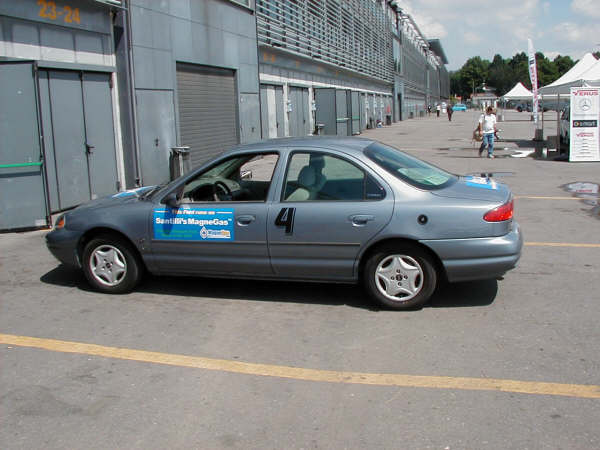
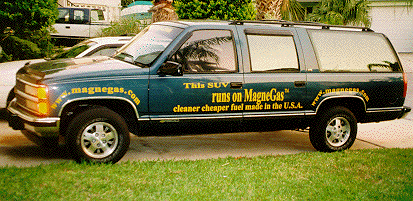
Figure 4.19. A view of some of the numerous cars prepared by
Santilli to operate on MagneGas. The picture from the top shows Santilli
with a Ferrari 308 GTSi 1981 he converted to operate on MagneGas while
being tested in 2001 at various Florida race tracks, including Sebring,
Moroso and others, to show that the car accelerates faster and revs
higher than the same car running on gasoline due to the high octane of
MagneGas (about 130), the lower temperature of the combustion exhaust
(about 30% lower) due to about 50% of water vapor in the same exhaust,
and other factors. The middle picture shows a Ford Contour 1999 bifuel
(namely, operating on gasoline and natural gas, with a switch on the
dash for the change of fuel), operated on MagneGas in lieu of natural
gas without any change, while being tested at the Race Track in Monza,
Italy, in June 2008. The bottom picture shows a Chevrolet Suburban 1994
produced for the sole operation on gasoline, with the additional
equipment needed to operate on MagneGas that is daily used by Santilli,
gasoline being used for refueling when MagneGas is
depleted.
As Santilli puts it: During WWII, Germany had no major petroleum
reserves and synthesized most of its fuel in both their gaseous and
liquid forms for use in cars, tanks as well as airplanes. In fact, the
catalytic liquefaction of gaseous fuels, today called Fisher-Tropsch,
was discovered in Germany during that period. A country synthesizing its
fuels to fight the rest of the world some sixty years ago is
incontrovertible evidence that, today, some sixty years later, our
dependence on petroleum fuels is a purely political occurrence, since
there is no possible or otherwise credible doubt on the technical
capability today by any country achieving fuel independence.
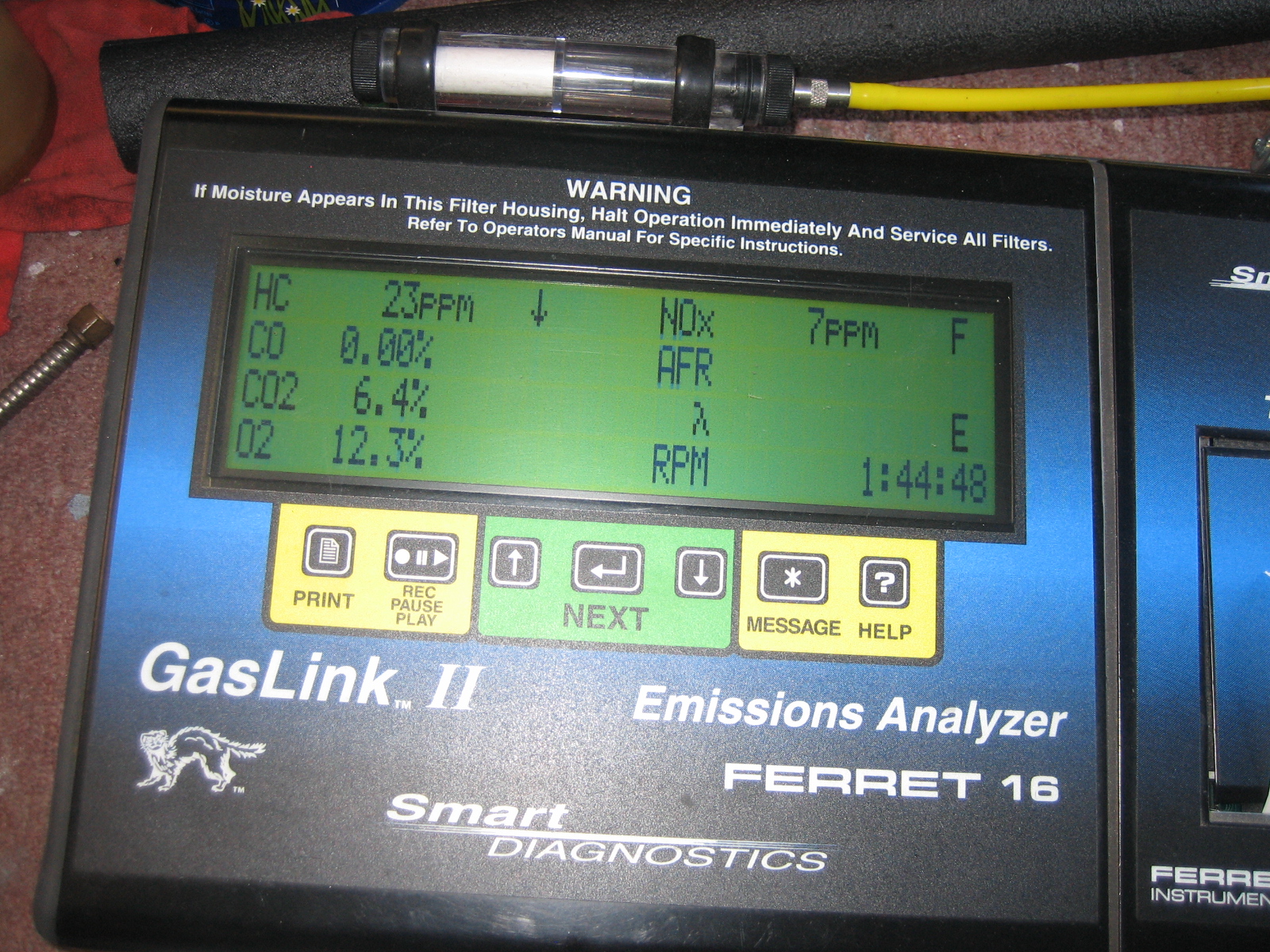
Figure 4.20. A picture of the reading of an exhaust analyzer
showing the combustion exhaust of the cars of the preceding figure, the
exhaust being sensed at the manifold, thus prior to the catalytic
converter. The data show the main characteristic of MagneGas of being a
fuel with complete combustion, thus without contaminants in the
exhaust. In fact, MagneGas exhaust is composed of about 50% water vapor,
12% to 14% breathable oxygen, 5% to 7% carbon dioxide, and the rest is
given by atmospheric gases. We should note that the detected HC
originate from the seepage of engine oil through the piston rings in a
rather old car, since MagneGas is produced at 10,000 degrees C at which
temperature no HC can survive. Similarly, CO is produced by the
combustion of fossil fuels, while CO is a fuel component for MagneGas.
Therefore, any presence of CO in the MagneGas exhaust is equivalent to
the presence of gasoline in the exhaust of a gasoline operated car,
namely, both are evidence of lack of proper combustion (for detail see
the quoted specialized literature).
4.4D. Industrial realization of the HHO fuel (2006)
Another fuel with a magnecular structure studied in detail by Santilli
is that produced via a special electrolyzer that turns distilled water
into a very powerful, combustible, and gaseous form for which he
introduced the chemical name of HHO. Prior to Santilli's studies,
a similar gas was known under the name of Brown gas but referred
to a perfect stochiometric mixture of 2/3 ordinary hydrogen and 1/3
ordinary oxygen gases.
However, Santilli showed that a 70-30 mixture of H2 and
O2 does not possess the special features of the HHO gas,
such as that of instantaneously melting tungsten and bricks at flame
contact. Hence, he conducted systematic experimental measurements
establishing that at least a percentage of the HHO gas has a magnecular
structure. Such a percentage is then crucial for the stability of the
gas, e.g., to avoid the perfect stochiometric ratio of hydrogen and
oxygen for selfcom,bustion. The results of the studies have been
reported in the historical paper (we cannot possibly review here for
brevity)
A new gaseous and combustion form of water
R. M. Santilli,
Intern. J. Hydrogen Energy Vol. 31, pages 1113-1128 (2006)
It should be stressed that, due to two years of delays between the date
of acceptance of the above paper and that of its publication, a
considerable confusion resulted and the above quoted printed version
is NOT the final version approved by Santilli but that of uncorrected
galleys with several garblings such as those in the symbols as well as
misprints. For the correct version, we refer the reader to the
monograph
The New Fuels with Magnecular Structure
Ruggero Maria Santilli
International Academic Press (2008)
200 pages
Italian translation by Giovanna Bonfanti and Michele Sacerdoti,
published by Editori Riuniti, Roma, Italy, and available at
I Nuovi Carburanti con Struttura Magnecolare
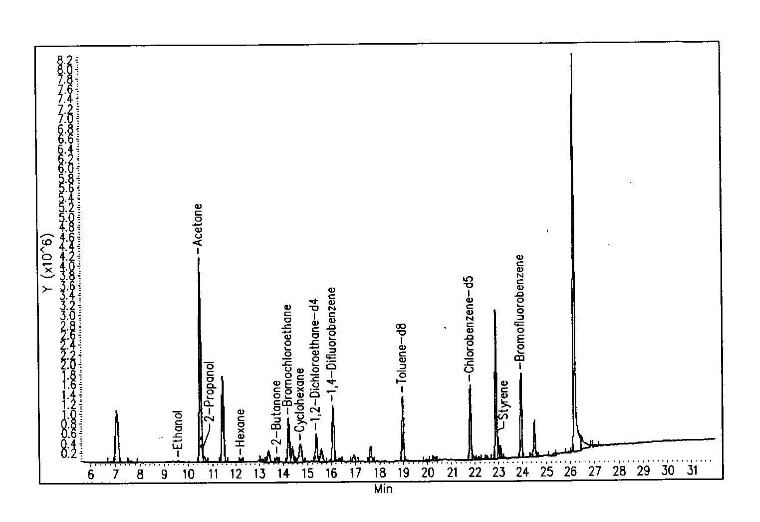
Figure 4.21. Experimental evidence of the magnecular structure
of the HHO gas: a printout of the blank following completion of the
scans with a GC-MS and removal of the gas from the instrument. For all
conventional molecular gases, the black solely shows background data
none in macroscopic percentage. By contrast, the blank of this figure is
essentially similar to the ordinary scan under the precautions indicated
in Section 4.3 (sufficiently large feeding line, low column
temperature, longest possible elusion time, etc.). Since the HHO gas was
removed from the instrument, the above printout establishes the
magnecular character of the gas via its anomalous adhesion to the walls
of the instrument. Additionally, the scan shows a number of species in
macroscopic percentage other than H2 and O2 that
can only be explained as magnecular clusters comprising combinations of
H or O, HO, H2, O2, and H2O, since
the gas originates from distilled water.
The research herein considered was conducted by Santilli as a scientific
consultant of the American company Hydrogen Technology Applications,
Inc. which company is the owner of all intellectual rights and is
currently producing and selling the HHO gas on a world wide basis under
the commercial name of AquygenTM. More details can be
obtained from the website
Hydrogen Technology Applications, Inc.
4.4E. Industrial realization of MagneHydrogen fuel (2003)
Santilli has been very supporting of hydrogen as a fuel to such an
extent that he dedicated years of research to the field. In particular,
he supports the environmentally acceptable production and combustion of
hydrogen consisting of:
1) Hydrogen should be produced via clean renewable sources of
electricity, such as those of wind, solar of hydro-origin;
2) The clean electricity is used for the electrolytic separation of
water; and, above all,
3) The oxygen produced in the process is released in the atmosphere so
that the combustion of hydrogen reacquires said oxygen by mantaining
unchanged the oxygen balance in our planet.
In reality, the current production and use of hydrogen is dramatically
different than the above sound environmental lines, by causing the
following serious environmental problems:
A. Hydrogen is generally produced from electrolytic separation of water
via currently available, environmentally unacceptable sources of
electricity. Alternatively, hydrogen is produced via the reformation of
fossil fuels that notoriously cause a pollution bigger than that caused
by gasoline combustion. Since the energy needed for hydrogen production
is bigger than its energy content, under the condition here considered
there is no real environmental or other gain in the use of hydrogen as
fuel.
B. In case hydrogen is produced from the electrolytic separation of
water, the produced oxygen is captured and sold for various scopes,
rather then released in the atmosphere, in which case the oxygen balance
in our plan et is lost at the time of oxygen combustion. For instance,
when oxygen is sold for metal cutting, it is turned into iron oxides.
Consequently, the combustion of hydrogen, whether in an engine or a fuel
cell, causes oxygen depletion (Section 4.4A), namely, the
permanent removal of breathable oxygen from our planet and its
conversion into water vapor.
C. Assuming that the above environmental aspects are solved, hydrogen
remains with serious additional problems, such as that of seepage
through the walls of containers due to its extremely small size, thus
causing known storage problems.
D. Hydrogen is the ligthest gas in nature. Therefore, when released in
the atmosphere due to seepage or other reasons, it rises very rapidly to
the ozone layer where it causes the additional environmental problem
called ozone depletion, namely, the permanent removal of ozone
from our atmosphere with consequential increase of skin and other forms
of cancer, due to the very fast chemical reaction (that has no
equivalent for other fuels and their exhaust)
(4.31) H2 + O3 → H2O +
O2
E. Additionally, hydrogen has a limited amount of energy content, thus
causing known storage problems to reach a desired range. In fact,
hydrogen has 300 BTU/scf., while gasoline has about 110,000 BTU/g.
Consequently, the Gasoline Gallon Equivalent (GGE) for hydrogen is
110,000/300 = 360 scf. It then follows that to reach the same range of a
tank with 30 gallons of gasoline, there is the need of 30 x 360 = 10,800
scf, namely, a volume of hydrogen so large that it cannot be effectively
stored in a car. This limitation has requested the cryogenic
liquefaction of hydrogen by some automakers, which liquefaction renders
the cost of hydrogen simply prohibitive for the foreseeable future since
hydrogen liquefies close to the absolute zeros degree temperature.
Additionally, hydrogen must be continuously maintained at the liquid
state irrespective of whether the car is used or not, thus causing
additional costs as well as danger in the event the on board cryogenic
system fails, since in this case there is an explosive transition from
the liquid to the gas form due to the very fast change of volume, rather
than combustion.
With his typical research style, Santilli addressed systematically all
the above problems and searched for their solution in support of
hydrogen as a fuel. Firstly, he developed the MagneGas Technology also
in support of hydrogen since MagneGas contains a minimum of 60 %
hydrogen in a mixture with other gases, thus being of easy
separation via membranes or other separation processes. This new form of
hydrogen production has numerous advantages over conventional producton,
such as:
I) An acceptable energy efficiency, since the energy needed for
hydrogen production is smaller than its energy content thanks to the
very high efficiency of hadronic reactors, thus alleviating the use of
environmentally unacceptable electricity for hydrogen production;
II) A significant reduction or elimination of oxygen depletion,
because MagneGas is rich of oxygen originating from liquids,
rather than from the atmosphere; and
III) A major reduction or elimination of the ozone depletion,
because the hydrogen produced from MagneGas has no seepage due to its
magnecular structure that seals the walls of all containers via layers
of atoms bonded by magnetic induction, as established by various tests.
Additionally, Santilli developed yet another fuel with a magnecular
structure under the name of MagneHydrogen, today known under the
chemical symbol MH. The objective was to avoid the cryogenic
liquefaction of hydrogen via the increase of its specific weight
because, in the event the specific weight of hydrogen can be increased
by a factor of 3, the "heavy" species of MH would reach an energy
content equivalent to that of natural gas, thus avoiding any need for
cryogenic liquefaction, with evident benefits for the hydrogen industry,
such as dramatically reduced costs, increased range, etc.
As it is well known, there is no possibility of increasing the specific
weight of hydrogen under a valence bond because valence electrons couple
in pairs, resulting in the conventional molecular structure
H2 = H-H with specific weight 2.016 amu. By contrast,
magnecular bonds have no theoretical limit in the number of bonded
atoms, the limit being set by the temperature and other conditions. It
is then evident that the only possibility for increasing the specific
weight of hydrogen is that via magnecular bonds, hence the name of
MagneHydrogen.
Santilli also searched for means to produce a form of MagneHydrogen with
specific weight bigger than 2.016 amu. Following various trials and
errors, he developed a process consisting in passing MagneGas through a
zeolite selected for hydrogen purification; collection the hydrogen
released; and then passing it again at pressure through the same zeolite
seven consecutive times. The species of hydrogen produced in this way
resulted to have about seven times the specific weight of
conventional hydrogen (see Figure 4.22 for more details).
The research herein outlined was conducted by Santilli as scientific
advisor of the U. S. corporation Clean Energies Tech, Inc. that
is the sole owner of all intellectual rights. A vast industrial effort
is under way at this writing (Spring 2009) to organize the industrial
production of MagneHydrogen.
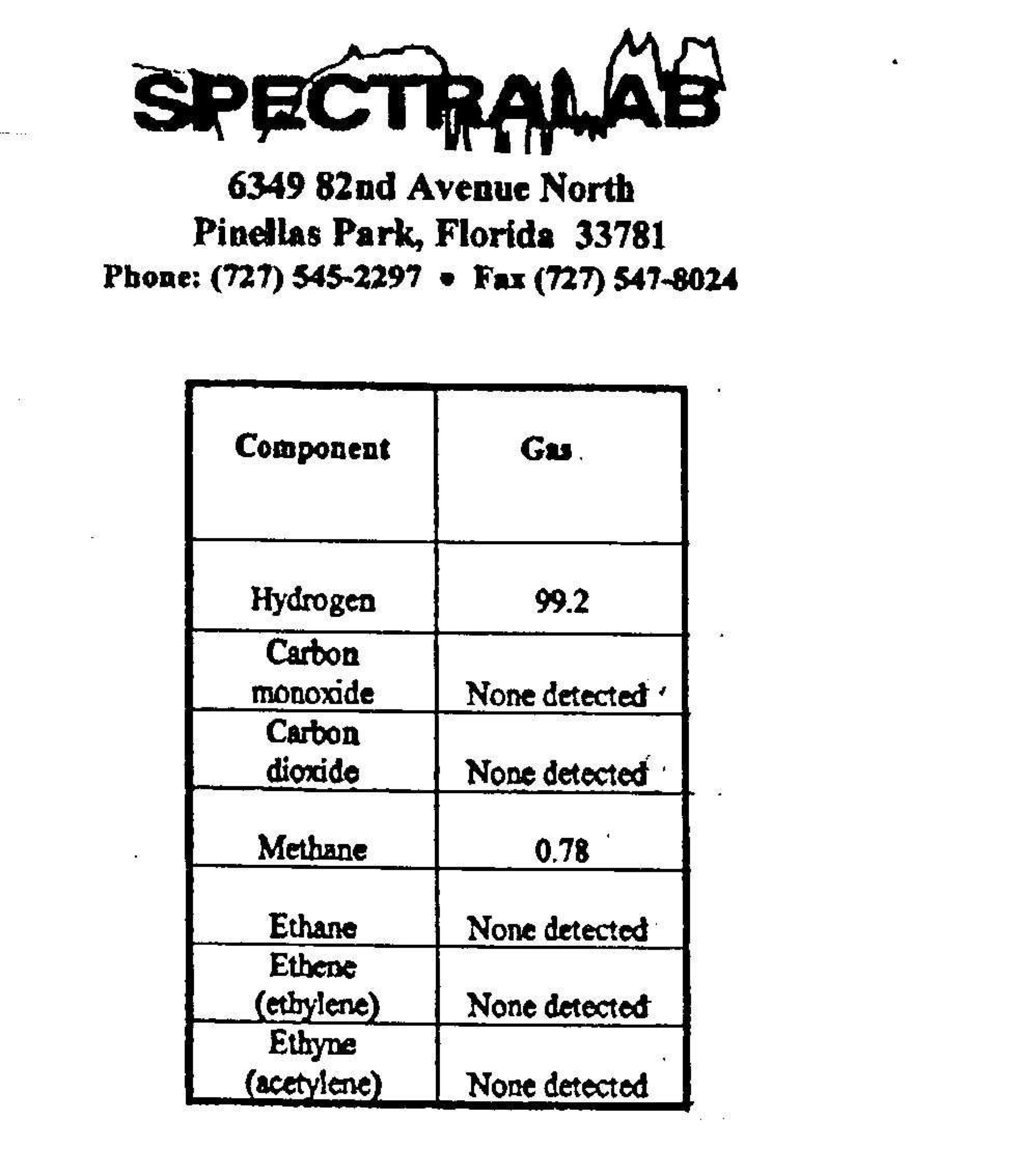
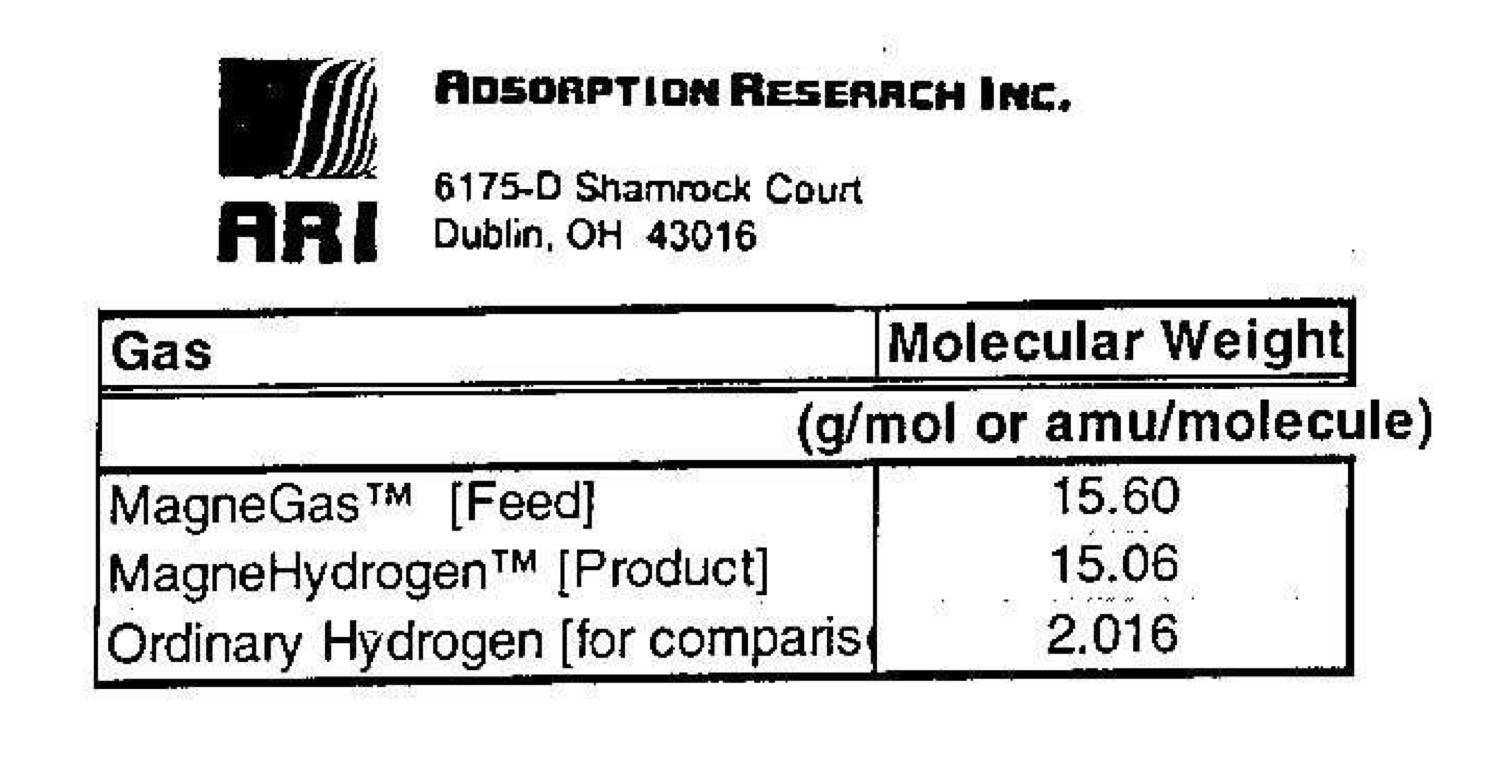
Figure 4.22. The measurements of the new species of
MagneHydrogen (MH) produced under Santilli's directions by independent
U. S. laboratories, whose directors released signed statements that have
been included in the historical paper of 2003 quoted below. As one can
see, the top measurements indicates that the species is composed of 99.2
% hydrogen while having the specific weight of 15.06 amu, that is, 7.4
times that of hydrogen. This evidence seals in a final and
incontrovertible way the existence of Santilli magnecules, by
disqualifying as nonscientific any theoretical or theological doubt
since only a basically new species, that is, a species with a basically
new bond other than the valence, can provide a credible representation
of the results.
To clarify the discovery, the hydrogen collected following the first
passage of MagneGas through the zeolite is expected to have indeed a
magnecular structure, but with a minimal increase of the specific weight
over that of the hydrogen. The subsequent passages of the species
through the same zeolite essentially cause the accumulation under
pressure of polarized hydrogen clusters along opposite polarities
North-South-North-South-..., resulting in a sequence of magnecular
clusters of the type
(4.32) MH1 = H,
(4.33) MH2 = H-H + HxH,
(4.34) MH3 = (H-H)xH + HxHxH,
(4.35) MH4 = (H-H)x(H-H) + Hx(H-H)xH + HxHxHxH,
etc.
whose main limitation is that of their breakdown due to collisions
caused by temperature.
The main reference of the discovery of MagneHydrogen is the additional
historical paper of 2003 whose pdf file contains copies of the signed
laboratory reports
The novel magnecular species of hydrogen and oxygen
with increased specific weight and energy content
R. M. Santilli,
Intern. J. Hydrogen Energy Vol. 28, 177-196 (2003)
as well as the monographs previously quoted.
4.4F. Molecular and magnecular combustions (1998)
Yet another branch of quantum chemistry that was never accepted by
Santilli as of final character is that pertaining to conventional
combustions, with reference to statements, e.g., that the combustion of
hydrogen and oxygen yields 57.5 Kcal/mole,
(4.36) H2 + O2/2 → H2O
+ 57,5 Kcal/mole.
Santilli's argument is that the two H-atoms are widely and permanently
separated in the H2O= H-O-H molecule, as clearly shown in
Figure 4.7. Therefore, he argues that the combustion of hydrogen and
oxygen releases much more energy than 57.5 Kcal/mole, the biggest
portion of which is used by nature to separate the H2 = H-H
and the O2 = O-O molecules, and the measured amount of 57.5
Kcal/mole is merely the small final energy residue.
In fact, we have the following well established and known separation
energies
(4.37) H2 → H + H - 104.2 Kcal/mole,
(4.38) O2/2 → (O + O)/2 - 119.1/2 Kcal/mole
Consequently, in Santilli's view, the belief that the combustion of
hydrogen and oxygen solely produce 57.5 Kcal/mole violates the sacred
principle of conservation of the energy since separations (4.37) and
(4.38) would then occur by academic fiat and not via physical or
chemical laws.
Santilli argues that, as a necessary condition to verify the principle
of conservation of the energy, the combustion of hydrogen and oxygen
must produce 221.25 Kcal/mole (rather than the claimed 57.5 Kcal/mole)
so as to provide the energy necessary for the separation of
H2 and O2 plus the 57.5 Kcal/mole residue,
according to the combustion law for molecular hydrogen and oxygen
(4.39) H2 + O2/2 → (H + H) + (O +
O)/2 + 221.25 Kcal/mole - 104.2 Kcal/mole - 59.5 →
→ H2O + 57.5 Kcal/mole.
Once the real combustion law has been understood, it is easy to see the
environmental and industrial importance of Santilli's fuels with
magnecular structure because they contain individual atoms under a bond
weaker than the valence bond. Therefore, magnecular fuels
yield an energy output greater than that of molecular fuels with the
same of atoms. To clarify these new combustions notions,
Santilli has introduced the following definitions:
MOLECULAR COMBUSTION: is that for fuels whose atoms are entirely
under a molecular bond, such as hydrogen. methane, gasoline, etc.
MAGNECULAR COMBUSTION: is that for fuels whose atoms are at least in
part, under a magnecular bond, and the rest under a molecular bond, such
as MagneGas, HHO, MagneHydrogen and others.
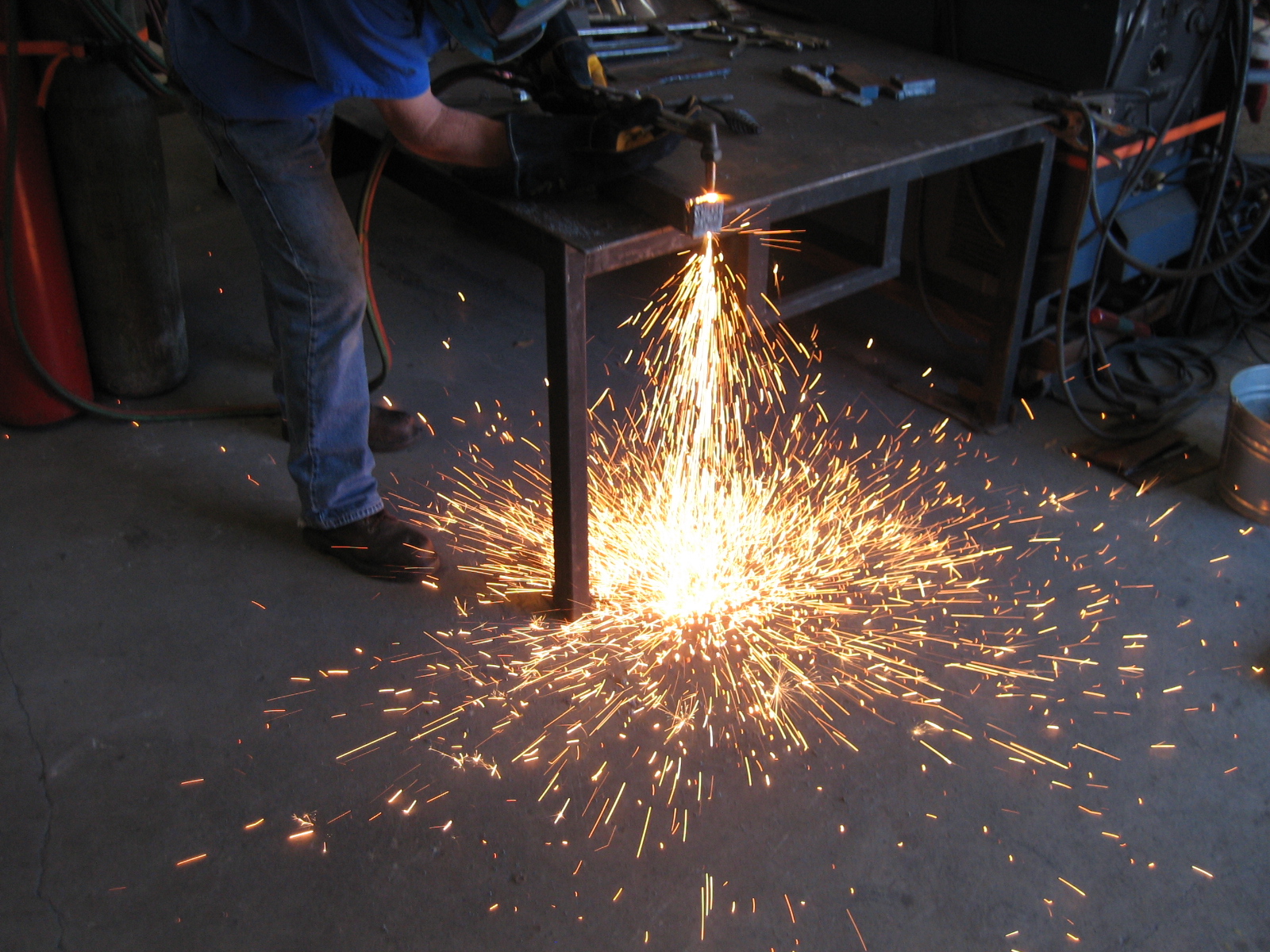
Figure 4.23. A picture of MagneGas produced from distilled
water with 70% H-atoms, 30% O-atoms and 30% C-atoms that cuts a 2" (5
cm) thick metal place at double the speed of acetylene. The belief that
said MagneGas has a conventional molecular structure would imply the gas
to have 236 BTU/scf, while acetylene possess 2,400 BTU/scf, thus
exiting the boundaries of science in favor of theologies. The sole
possible quantitative interpretation is that the MagneGas here
considered has Santilli's magnecular structure with a percentage of
atoms being weakly bounded, thus ready for combustion, plus toroidal
polarizations of the orbitals discussed in the text.
The superior energy output of magnecular fuels with respect to molecular
fuels with the same atomic composition is beyond any possible or
otherwise credible doubt. Consider, for instance, MagneGas produced from
distilled water that contains 70% H-atoms, 30% O-atoms and 30% C-atoms,
plus impurities in ppm here inessential. Recall that H2
contains about 300 BTU/scf, while CO contains about 89 BTU/scf.
Consequently, the fuel with conventional chemical composition of 70%
H2 and 30% CO contains (0.7 x 300 + 0.3 x 89) BTU/scf = 236
BTU/scf. It is beyond doubt that such a molecular fuel cannot possibly
cut metal faster than acetylene, as any skeptic is requested to verify
experimentally, as Santilli does, prior to venturing nonscientific
theologies.
By comparison, the gas with the same atomic structure, but with a
magnecular structure as currently in production and sale, cuts metal at
double the speed of acetylene that contains 2,400 BTU/scf. Any continued
belief on the dominance of the valence bond for all possible substances
existing in the universe until the end of time, causes the existing of
quantitative science and the passage to theologies. The only
possible explanation of the energy output of MagneGas being bigger than
acetylene is that via Santilli's principles of combustion based on the
presence individual atros under a wek bond..
A fully similar situation occurs for the HHO gas that, according to
quantum chemistry, should contain 210 BTU/scf, namely, an output of
energy immensely insufficient for the instantaneous melting of tungsten
and bricks. The same excess energy output occurs for MagneHydrogen and
all gases with Santilli magnecular structure.
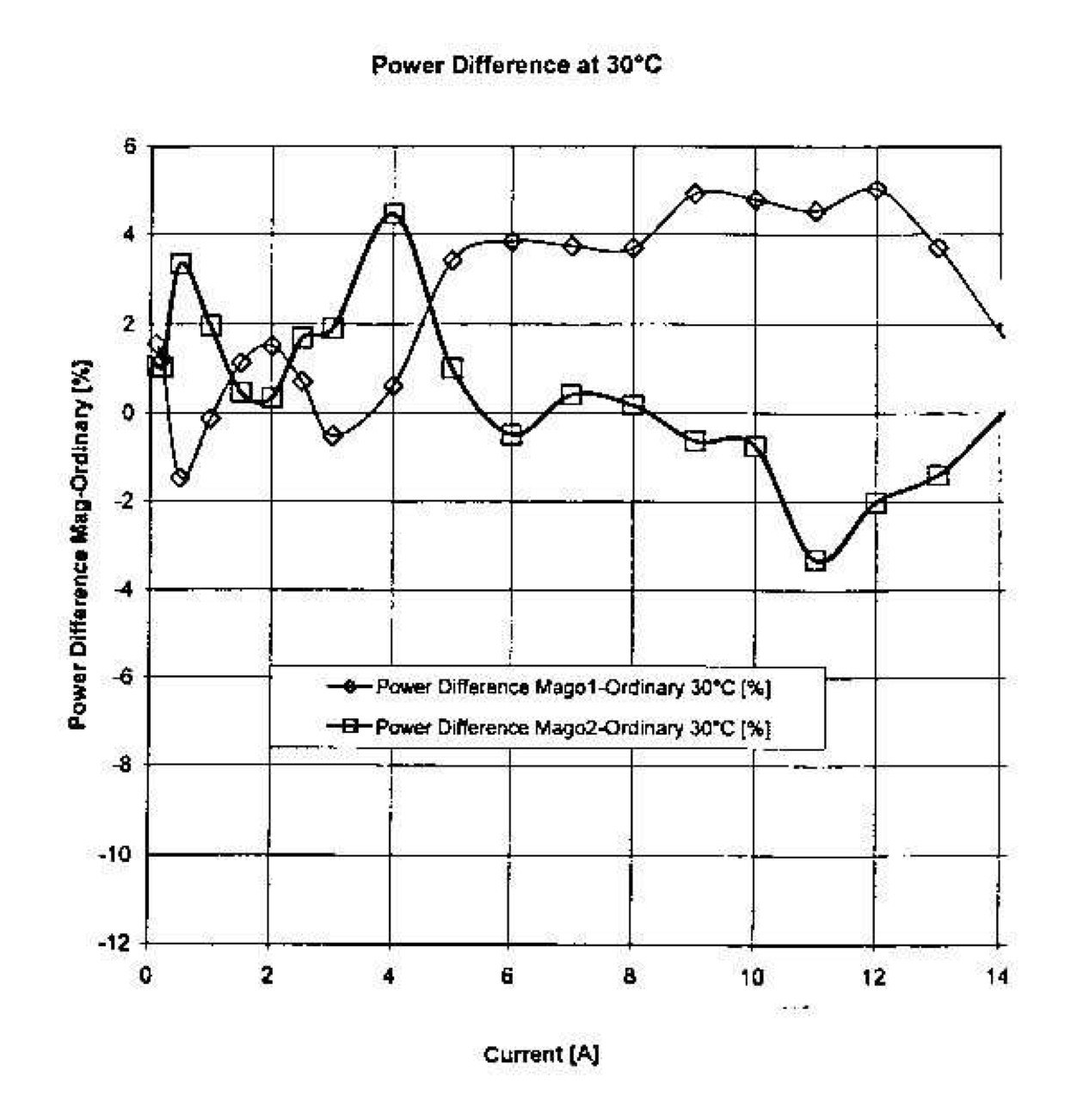
Figure 4.24. In the year 2000, Santilli tested hydrogen
separated from MagneGas in an experimental fuel cell and obtained the
increase of power output shown in the above picture. Similar increases
of power were obtained via a conventional hydrogen treated with a
special PlasmaArcFlow gaseous hadronic reactor (patented and
international patnts pending). The principle of the increased power is
precisely that of Santilli's magnecular combustion and it is due to the
substantial decrease of energy lost in molecular separation, thus
yielding a greater energy output. The above tests were halted by
Santilli because of extreme lack of interest by all fuel cell
industries and laboratories he contacted, due to the widely adopted
belief in academia and industry according to which, as Santilli's puts
it: "Novelty does not exist; novelty cannot exist; and if novelty is
proposed for test, it is a fraud." At any rate, the above scan as well
as numerous others have been available in the internet since 2000 and no
fuel fell industry has shown to date any interest whatsoever in even
considering the verification or denial of a substantial increase of
their energy output via the use of Santilli magnecular fuel, nowadays
widely known in academia and the industry. Because of this lack of
interest, Santilli left the fuel cell industry essentially to its own
state, that of the past century.
To initiate a quantitative analysis, A. K. Aringazin and R. M. Santilli
assume that the H-atom is bonded magnecular with a yet unknown value
of s Kcal/mole. Therefore, we have the following data for the combustion
of MH2 = HxH in oxygen
(4.40) HxH → H + H - s Kcal/mole,
(4.41) O2/2 → (O + O)/2 - 59.55
Kcal/mole,
(4.42) HxH + O2/2 → H2O + (161.7 - s)
Kcal/mole,
where one should keep in mind that the value s = 0 is expected at the
combustion temperature.
It is easy to see that all mixtures of molecular H2 and
magnecular MH2 yield a combustion energy output bigger than
57.5 Kcal/mole. As an example, a mixture of 10% MH2 and 90%
H2 would yield (62.71 - s) Kcal/mole. For more details, we
refer the interested chemist to
Rudiments of molecular and magnecular combustions
A. K. Aringazin and R. M. Santilli,
Preprint of the Eurasian University (2009) to be published
It should be stressed that the studies on Santilli's magnecular
combustion are just at their infancy. For instance, Santilli stresses
that the sole energy output of reactions (4.39), (4.42) remains
basically insufficient to explain quantitatively how MagneGas cuts metal
faster than acetylene. This requires additional studies, not only on the
presence of weakly bounded atoms, but also that such atoms have a
toroidal rather than spherical polarization, since solely the latter may
explain the very fast penetration of hydrogen within the deep layers of
steel, with consequential melting and cutting much faster than acetylene
whose molecules remain at the surface due to their size.
4.5. SANTILLI DISCOVERY IN BIOLOGY
4.5A. Historical notes
There is no doubt that Santilli achieved his most advanced discoveries
in biology whose foundations are outlined in the monograph
"Isotopic, Genotopic and Hyperstructural Methods in Theoretical
Biology"
R. M. Santilli,
Ukraine Academy of Sciences (1997)
In this section we shall attempt a conceptual review of these advances
with the understanding that such a task is faced with serious
difficulties due to the complexity of the conceptions as well as of the
mathematics used for their quantitative treatment. Another difficulty of
this review is that Santilli has not published a number of advances in
biology that have been made available to us as notes not completed for
publication, although we have been told that the completion of these
papers and their publication are expected in the near future.
On historical grounds, Santilli has been one of the leading scientists
to establish the basic insufficiencies of quantum mechanics and
chemistry for biological structures. As he puts it: Any belief of
conducting serious quantitative studies of biological structures with
quantum mechanics and chemistry raises issues of scientific ethics and
accountability because first year graduate students know that said
theories predict biological entities to be perfectly rigid, perfectly
eternal and with no reproductive capacity. Therefore, the selection of
the applicable "generalization" of quantum mechanics and chemistry
should indeed be subject of scientific debates, but not its need.
It is our opinion that the year 1997 of publication of the above quoted
monograph will be remembered by ethically sound scholars as signaling
the transition from rudimentary studies in biology to fundamentally new
and dramatically more complex scientific vistas with truly vast horizons
and outcome beyond our predictive capacity.
In closing, Santilli has requested to remember Prof. Bakunin, the lady
who taught him organic chemistry at the University of Naples, Italy,
during his undergraduate studies in the 1950s, since his interest in
biology originated from her teaching.
4.5B. Deformability, irreversibility, and multi-valuedness of
biological structures.
The first and perhaps most important contribution by Santilli to biology
has been the identification of the following main characteristics of
biological structures, which identification sets the basis for the
discovery of the generalized mathematics needed for quantitative
treatments:
DEFORMABILITY. Santilli often initiates lectures in the field by
squeezing in front of the audience a small rubber ball and stating:
This simple deformation of a rubber ball is incompatible with special
relativity and quantum mechanics because it violates their central
pillar, the rotational symmetry, from which there is a consequential
inevitable violation of the Lorentz symmetry. He then moves his
fingers and states: A main feature of biological structure is
deformability requiring a dramatic departure from the 20th century
theories. In the absence of a theory representing deformability from its
main axioms, we know today that no invariant quantitative treatment is
possible..
IRREVERSIBILITY. Santilli then projects in the lecture screen a
representative biological event, such as the birth and death of a
flower, and states: Yet another main feature of biological
structures is their irreversibility over time. This feature, alone, is
sufficient to rule out all dominant theories of the 20th century, since
they were conceived , developed and tested to represent systems
reversible over time, such as the structure of nuclei, atoms and
molecules. Again, without methods that are irreversible in their basic
axioms, we merely have the illusion of quantitative studies in
biology.
MULTI-VALUEDNESS. Santilli then recalls the studies by the
Australian biologist Chris R. Illert who showed that a three-dimensional
Euclidean space can indeed represent all shapes of seashells, but
the computerized use of the same space to represent the growth of
seashells causes the latter first to grow in a deformed fashion and then
crack. Santilli then states: The basic axioms of the Euclidean space
at the foundation of the 20th century theories not only provide an
axiomatization of perfect rigidity and reversibility, but also the
representation axes are single valued, that is, they grant one single
value for each point. By comparison, Illert has shown that a more
accurate representation of the growth of seashells occurs via the use of
a six-dimensional space, that is, a space in which each axis is
doubled. It is evident that, when passing from the relatively
"simple" seashells to complex bio;logical structures such as a DNA, the
number of dimensions needed for a quantitative treatment may become
beyond our intuitional capacities. For more details, one should consult
Illert's contributions in the monograph
"Foundation of Theoretical Conchology"
C. R. Illert and R. M. Santilli,
Hadronic Press (1995)
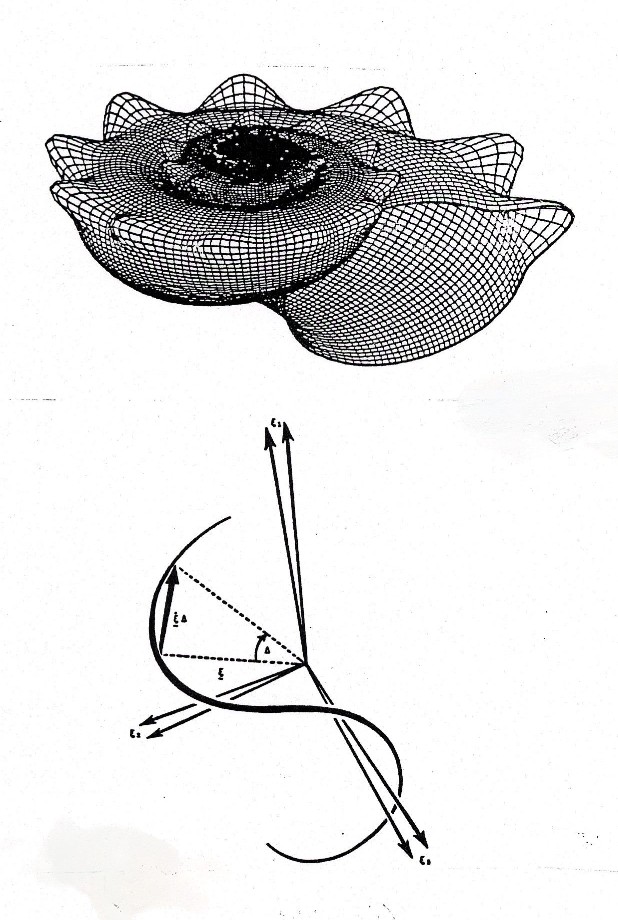
Figure 4.25. A conceptual rendering of Illert's representation
of seashells growth with the doubling of the Euclidean reference
axes.
4.5C. Representation of biological structures via Santilli's
deformable, irreversible and multi-valued hypermathematics
Illert's doubling (in 1995) of the three Euclidean axes for the
representation of seashells growth motivated the discovery in 1996 by
Santilli of hypermathematics presented in the historical mathematical
memoir
Nonlocal-integral isotopies of
differential calculus,
mechanics and geometries
R. M. Santilli, Rendiconti Circolo Matematico Palermo, Suppl. Vol.
42, 7-82 (1996).
and summarized in Chapter 2. As Santilli's put's it: When we observe
a seashell in our hands, we can fully perceive its growth via our three
Eustachian lobes. The sole way to reconcile the six-dimensionality of
seashell growth with our three-dimensional sensory perception is via a
hyper-Euclidean space with three dimensions in which each axis is
two-valued. At the abstract, realization-free level, there is no
distinction between the two-valued hyper-Euclidean space and the
conventional space, thus allowing our senses to perceive the seashell
growth in three dimensions. By contrast, the use of a six-dimensional
space would lead to irreconcilable incompatibilities between the
mathematical representation and our perception of reality.
Additionally, the multi-valued hyperspace must be irreversible as a
necessary condition not to violate causality. This requirement can be
implemented via the adoption of Santilli's multi-valued
genospaces, as presented in the above quoted mathematical memoir and
outlined in Chapter 2. As the reader will recall, irreversibility is set
at the most primitive level, the basic hyperunits, that are different
for different directions of time, thus assuring lack of violation of
causality.
Once a multi-valued irreversible genospace is assumed as the carrier
space, the invariant representation of deformations follows from
Santilli's multi-valued hyperrotational symmetry, namely, the
isorotational symmetry at the foundation of isorelativity and hadronic
mechanics (Sections 3.10 and 3.11) in which the basic isounits are first
differentiated for motions forward and backward in time, and then they
are assumed to be multi-valued. The invariant representation of
deformability under multivaluedness then follows, jointly with the
bypassing of the Theorems of catastrophic Inconsistencies outlined in
Section 3.7.
A comprehensive study of the isorotational symmetry and related
isotrigonometry is available in the following monographs
"Elements of Hadronic Mechanics",
Vol. I: "Mathematical Foundations"
R. M. Santilli, Ukraine Academy of Sciences (1993),
"Elements of Hadronic Mechanics"
Vol. II: "Theoretical Foundations"
R. M. Santilli, Ukraine Academy of Sciences (1994)
The deformable, irreversible and multi-valued hyperrotational symmetry
is then achieved via the reformulation of isomathematics into the
hypermathematics outlined below.
We assume the reader is aware of the differences between Santilli's
hypermathematics and the conventional hyperstructures. Both
are multi-valued, but the former consists of a comprehensive,
multi-valued irreversible lifting of the entire mathematics, including
units, numbers, spaces, symmetries, algebras, geometries, topology,
etc., all based on conventional operations. By contrast,
hyperstructures are generally reversible over time, are based on
abstract hyperoperations and do not possess left and right units, under
which conditions there is no direct and consistent application to
experimental measurements.
For clarity, hypermathematics that can be outlined, separately, for
hypertimes and hyperspaces.
SANTILLI HYPERTIMES
The need for the representation of biological structures via Santilli
deformable, irreversible and multi-valued hypermathematics has been
confirmed by various different approaches. One of them is by identifying
the notion of time needed for biological structures. Illert has
shown that a necessary condition for a seashell to form
bifurcations is to master all directions of time that, contrary
to popular belief, are four, being given by: motions forward
and backward in future time and in past times.
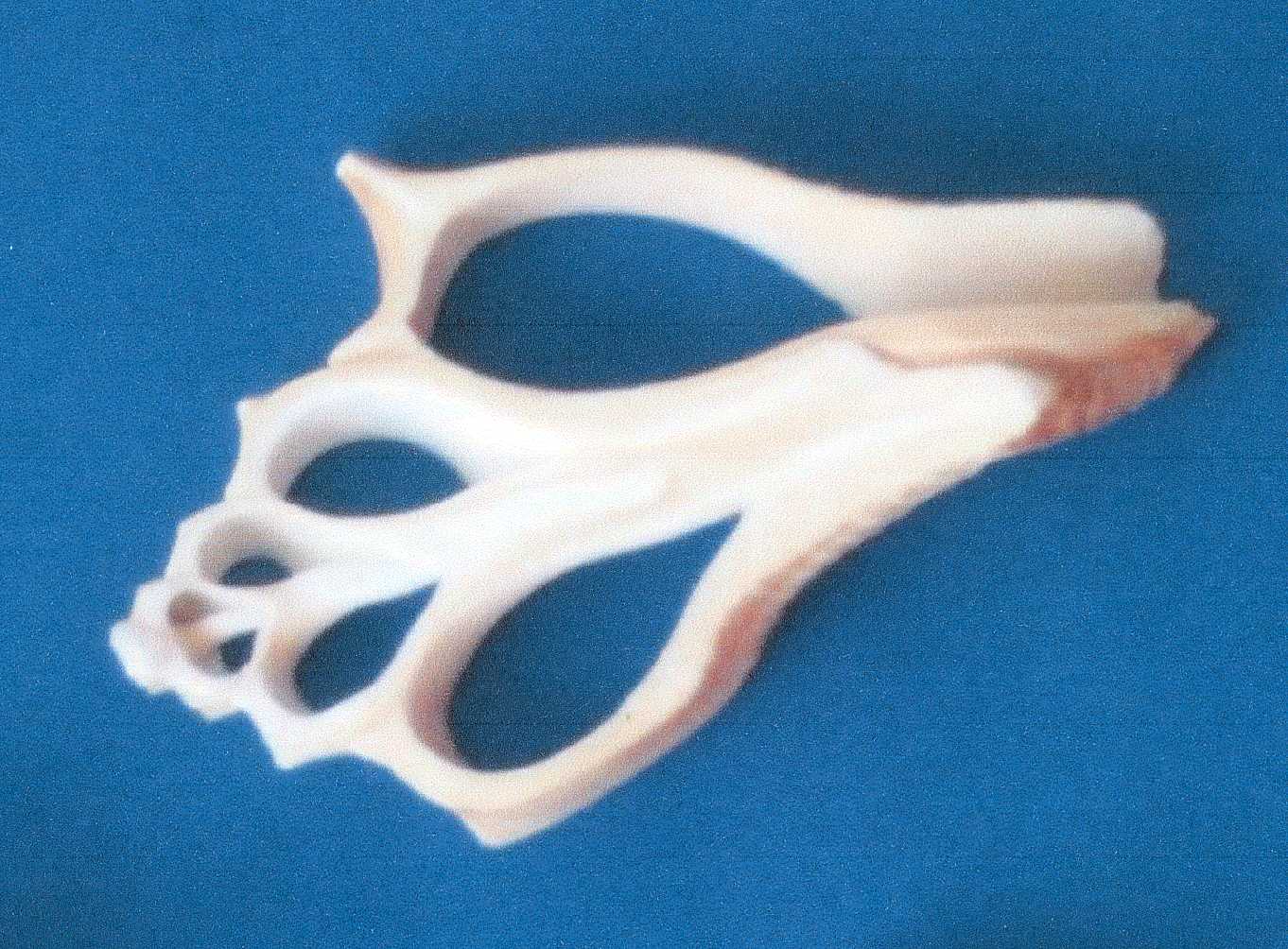
Figure 4.26. The slicing of a small seashell showing the
various bifurcations despite its small size. The notion of time needed
for a quantitative representation of the construction by the seashells
of all these bifurcations is so complex to be truly beyond human
comprehension. It can be represented via Santilli's hypertime consisting
of the ordered set of four motions in future and past time, each one
being multi-valued.
The sole known rigorous representation of the above occurrence is via
Santilli multi-valued hypermathematics and its isodual. In fact, the
conjugation of widespread use in the 20th century, time inversion, can
only represent the transition from motion forward to future times into
backward in past time (represented with upper "f" and "b",
respectively). The sole known way to achieve the remaining two
directions of time is via Santilli isoduality (2.9) (represented with an
upper "d"). We have in this way the following Santilli's four
different hypertimes
(4,43) t = {tf, tb, tfd,
tbd}, t
(4.44) tf: Motion forward in future times,
(4.45) tb: Motion backward in past times,
(4.46) tfd: Motion forward in past times,
(4.47) tbd: Motion backward in future times.
Eqs. (4.43)-(4,47) merely initiate the illustration of the complexities
of biological structures. In fact, Santilli's hypermathematics is based
on hyperunits, as shown in Chapter 2, that are generally different for
different times (as well as different space components), and we have the
following time hyperunits
(4.48) It = {Itf, Itb,
Itfd, Itbd},
Therefore, the four different times (4.43) are not measured with respect
to the conventional unit of time, say, 1 sec, but each hypertime is
measured with respect to its own hyperunit, by continuing to illustrate
the complexity of biological structures. In fact, the four hypertimes,
to be hypernumbers, must have the explicit structure
(4.49) tf = t1 Itf,
tb = - t2 Itb, tfd = -
t3 Itfd, tbd = t4
Itbd.
where tk, k = 1, 2, 3, 4, are conventional positive numbers.
The complexity of biological structures is further illustrated by the
fact that each hypertime and related hyperunit can be
multi-valued,
(4.50) It = { (Itf1,
Itf2, ...), (Itb1,
Itb2, ...),
(Itfd1, Itfd2, ...),
(Itbd1, Itbd2, ...)
}
But, each hypertime characterizes its own hyperspace. Thus, already at
this introductory level we see the need for four-values
hyperspaces, and we write,
(4.51) St = {Sf, Sb,
Sfd. Sbd},
each component being multi-valued. But we perceive the growth of a
seashell with our sensory perception based on a unique time evolution.
The sole known way to achieve compatibility between the multi-valued
mathematics needed for biology and our single-valued evolution in time
is that via Santilli hypertime since, at the abstract realization-free
level the multi-valued character disappears. Alternatively, we can say
that the abstract, realization-free axioms characterize our sensory
perception of time, while actual calculations are done with specific
multi-valued realizations.
More explicitly, Santilli states that the ordinary time t perceived by
us is realized into the four-fold times (4.43) and, correspondingly, our
time unit It = 1 sec is factorized into the four hyperunits
of Eq. (4.48), each one possibly being multi-valued as in Eqs. (4.50)
depending on the complexity of the case at hand. For the case of
Illert's seashells, the four hyperunits can be single-valued resulting
in the conventional four directions of time (4.44)-(4.47). However, for
more complex biological structures, each hyperunit can be multivalued,
resulting in eqs. (4.50).
In this way, we are naturally forced to distinguish the observer
time, which is our perception of time, and the intrinsic
time, which is of such a complexity as being outside our intuition
and solely representable with mathematical language.
As an illustration, consider only the forward hypertime. Its hyperunit
If can have positive-definite but arbitrarily small or
arbitrarily large values. Even though we perceive a seashell in our
hands with our observer's time, in its own internal perception the
seashell can be in the extreme past or the extreme future. The addition
of the remaining three hypertimes illustrates again the inability of our
limited mental capacity to understand the complexity of a relatively
"simple" biological structure such as a seashell, much bigger
complexities being expected for the DNA structure.
SANTILLI HYPERSPACES
Let us pass to the outline of Santilli's hyperspaces. Assume at the
abstract level a point P in Euclidean space with coordinates P = {x, y,
z} and realize the latter in the following multi-valued space
hypercoordinates (each set being ordered),
(4.52) P = {x, y, z} → { (x1, x2,
...), (y1, y2, ...) (z1, z2,
...) },
with corresponding space hyperunits
(4.53) Itot =
{ (I1x = 1/n21x,
I2x = 1/n22x, ...), (I1y =
1/n21y, I2y =
1/n22y, ...) (I1z =
1/n21z, I2z =
1/n22z, ...) },
and related hyperproduct as identified in Section 2.2C. The hyperline
element left invariant by the hyperrotational symmetry can be written
(4.54) r2 = x2 + y2 +
z2 →
→ r*2 = (x12
n21x + x22
n22x + ...) +
(y12 n21y +
y22 n22y + ...) +
(z12 n21z +
z22 n22z + ...),
which line element illustrates in a transparent way the deformability of
the original perfect sphere x2 + y2 +
z2. The universal invariance is then given by the
hyperrotational symmetry as indicated above.
We are regrettably forced to halt here our rudimentary review of
Santilli's hypermathematics to prevent excessive length. We limit
ourselves to stress, again, that the complexity of biological structures
is truly beyond human intuition, as illustrated by the hyperbox
of Figure 2.1 reproduced below under a new interpretation.
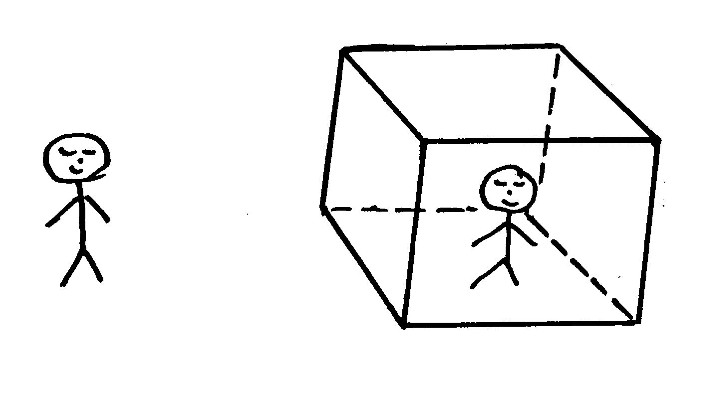
Figure 4.27: In Figure 2.1 we have described Santilli isobox,
while in this figure we describe the hyperbox consisting of a box
examined by two observers, an external observer in our time and space,
and an internal observer in hypertime and hyperspace. For the case of
the isobox, both observers are in a (3+1)-dimensional space, the main
differences being the perception of much different shapes in generally
different times. For the case of the hyperbox, the differences between
the exterior and interior observer become of truly difficult
understanding by the limited capacities of the human mind, and can
solely treated mathematically, since the exterior observer is in a
(3+1)-dimensional space, while the interior observer is in a space with
an unlimited number of folds branching into multi-dimensional
universes.
4.5D. Hypermolecules, hypermagnecules and hyperliquids
The origin of Santilli's prolific discoveries in so different fields is
his conviction that quantitative sciences will never admit final
descriptions, a limitation that he applied primarily to his own
advances. As an example, following the discovery of the isonumbers
(Section 2.2A) that, alone, would have assured his name in the history
of mathematics, Santilli identified their limitations and, in so doing,
discovered the genonumbers; then he identified their limitations and,
in so doing, discovered the hypernumbers; then he identified their
limitations and, in so doing, discovered the isodual numbers.
Santilli systematically applied this self-criticism to essentially all
his discoveries. Consider, for instance, the achievement t of the first
known quantitative representation of molecules and their valence
bond with an explicitly identified attractive valence force in complete
agreement with experimental data (section 4.2). That achievement, alone,
was sufficient to set his name in the history of chemistry.
Nevertheless, Santilli remained dissatisfied because he considered the
advance excessively limited with respect to the complexities of nature.
Consider, for instance, the water molecule. It is popularly believed
that such a molecule has one and only one representation, and that it is
the same whether it is in our atmosphere or part of a cell. Santilli
considers such a view rather arrogant because it assumes a final
knowledge of one of the most complex structures in the universe with
capabilities and feature simply beyond our imagination at this writing.
In reality, there are reasons to expect that, when all features of a
cell are taken into account, including its reproductive capacity, each
water molecule of a cell is in some form of communication with all the
remaining molecules of the same cell. Additional evidence indicates that
one water molecule of one cell may well be in some form of communication
with all other cells of a body, and so on.
The only quantitative way of initiating the study of such a complexity
is via hypermathematics. In this way, Santilli worked out in a paper
made available to the Foundation(and expected to be published soon for
uploading in pdf format when completed) his model of
hypermolecules essentially consisting in the reformulation of the
molecular models of Sections 4.2 via multi-valued hypermathematics.
Santilli then applied the same self-critical analysis to his
magnecules to discover, again, their excessive limitations for
biological structure. In this way, in an additional paper made available
to the Foundation and expected to be published, Santilli introduced his
hypermagnecules, namely, nonvalence bonds primarily due to
opposing magnetic (and electric) polarizations, each bond being
multivalued.
Santilli hyperliquid is, therefore, composed by hypermolecules
under hypermagnecular bonds by achieving in this way one of the most
complex structures known to the authors, not only for mathematical
treatment, but also because of the truly unlimited possibilities of
interconnections at a distance, as manifestly necessary for any
serious understanding of the complexities of bio;logical systems.
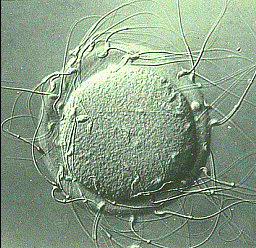
Figure 4.28: The fusion of two gametes to form a zygote and
initiate a new life. Santilli argues that the original two individual
gametes, generally classified as cells, have in reality an extremely
complex structure since they show a specific purpose, movements and
action. Hence, their cytoplasm and other components cannot be merely
made up of ordinary molecules with quantitatively unknown valence bond
and links into a liquid state via quantitatively unknown H-bridges.
Hence, he represents each individual genome via his notion of
hyperliquid. Additionally, Santilli argues that the two gametes cannot
be considered independent one from the other since they seek each other.
A representation of this interconnection at a distance is also permitted
by the notion of hyperliquid, since the latter allows, in principle, the
connection of one cell with all possible cells existing in the universe,
of course in a way inversely proportional to the square of the distance,
much along the fact that the wavepacket of one electron can be
considered to be null only at infinite distance.
4.5E. Deciphering the DNA code?
The deciphering of the code contained in a DeoxiribonNucleic Acid (DNA)
is, by far, the most cryptographic problem facing mankind. Santilli felt
repugnance to the idea that a code of such a complexity could be
understood with the ordinary numbers 1, 2, 3, ... dating back to
pre-biblical times. With the understanding that the achievement of a
solution will require centuries of studies, Santilli introduced his
multi-valued hypernumbers for the specific intent of initiating
quantitative studies on the DNA code.
The main argument is that the association of two atoms in a DNA can
produce an entire organ, such as the liver, with an extremely large
number of constituents. The association of two atoms A1 and
A1 in a DNA can be mathematically represented via the
multiplication. The multiplicity of the results of the original
association then leads, inevitably, to Santilli hypernumbers in which
the product of two elements can give rise to an ordered, but unlimited
number of results, e.g.,
(4.55) A1 x A1 = { 1.7684, ∫ f(r)dr,
745.344, log(p^er), .......}.
The capability by the hypernumbers of at least initiating the
deciphering of the DNA code is evident. Despite these evident
possibilities, Santilli call published in the historical 1997 monograph
"Isotopic, Genotopic and Hyperstructural Methods in Theoretical
Biology"
R. M. Santilli,
Ukraine Academy of Sciences (1997)
as well as in other papers, has remained unanswered by biologists,
perhaps due to their limitation to understand Santilli's mathematics.
The point is that, without an adequate advanced mathematics, biologists
merely have the illusions of advances in their field.
4.5F. Understanding the DNA structure?
Santilli never accepted as final the idea that the DNA has a molecular
structure, as stated in the best books in the field (see also wikipedia)
because excessive simplistic when compared to the complexities of the
structure considered. Therefore,he suggested that the DNA has a
magnecular structure, namely, it is characterized by atoms that, in
part, are under a molecular bond, and in part under a magnecular bond.
The hypothesis is strongly supported by the numerous unknown "H-bridges"
in the field, with Santilli magnecular bond with a clearly identified
attractive force.
Despite a clear advance over rather simplistic models in the literature,
Santilli remained dissatisfied with said magnecular structure because
basically insufficient to provide the extremely complex inter-relations
needed to explain the production of a large organism from a minute helix
of atoms.
In this way, he reached one of his most important notions, that that
the DNA has a hypermagnecular structure, as conceptually
indicated in the preceding sections. This essentially means the
conception of the DNA as being composed of atoms under hypermolecular
bonds that, in turn, are under a hypermagnecular bond.
A rather feverish research is ongoing at a number of corporations in the
U.S.A. and abroad. We regret the prohibition to report these studies
because of expected disruptions by academic chemists usually aimed at
halting the funding to suppress undesired advances.
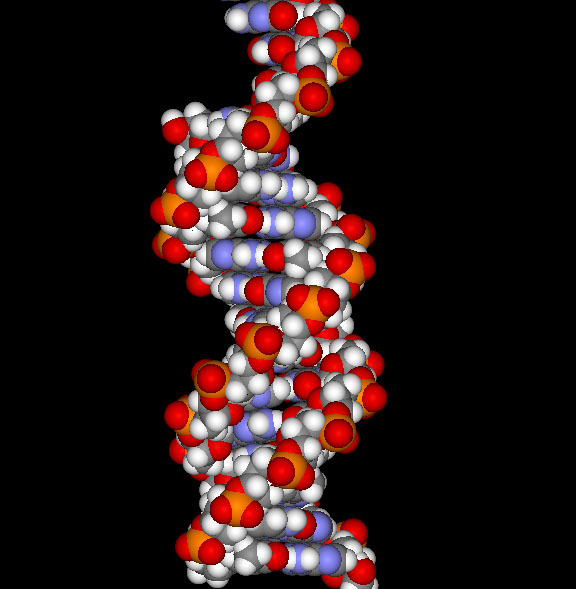
Figure 4.29: Santilli never accepted as final the idea that
the DNA is a "molecule" (see wikipedia and advanced references quoted
therein) for various reason. To begin, valence electrons can solely bond
in pairs under singlet couplings resulting in bosons with null spin
(Figure 4.2). A first year graduate student knows that no additional
spin 1/2 electron can be credibly bonded to a spin 0 valence electron
pair. Therefore, the belief that five hundred million atoms of a DNA
could be kept together by valence bonds caused the exiting of science in
favor of theology. The next possibility is that a DNA could be a
"liquid" since its molecules are admitted in the literature as being
bonded by "H-=bridges." However, this alternative hypothesis is faced
with gross inconsistencies, e.g., the doubling of cells, the fusion of
gamedes, and other basic events deviate from the prediction of liquid
structures (e.g., via surface tension), again, to such an extent of
causing the exiting of science in favor of theology. Santilli's main
stand is that the structure of the DNA is immensely beyond all 20th
century knowledge of chemistry. To initiate scientific, that is,
quantitative studies, Santilli introduced first the hypothesis that the
DNA has a magnecular structure so as to replaced the nomenclature of
"H-bridges" with equations and actual attractive forces discussed in
Section 4.3E. However, the hypothesis soon turned out to be
insufficient, e.g., because of the inability to represent cell
interconnections at a distance (Figure 4.28). Consequently, Santilli
formulated the broader hypothesis that the DNA has the structure of a
hypermagnecule. A rather feverish research is going on at U. S. and
foreign companies (rather than academia) along a hierarchy of
hypermagnecular structures of increasing complexity, the first one being
that bonding together hypermolecules, the second one at the level of
chromosomes, and so on. It is regrettable that the current condition of
scientific ethics in academic chemistry prevents the disclosure of these
industrial studies.
4.5G. A future new cure for cancer?
Self-appointed pseudo-scientist generally dub as "semantic" basic
advances without any serious study because beyond their comprehension.
This is also the case for Santilli's discoveries in biology, although by
a rapidly decreasing number of academicians. The problem for said
pseudo-scientist is that all Santilli discoveries have concrete
applications under development by the industry and certainly not by
academia, with due exceptions, because of the novelty.
Santilli introduced the notion of magneliquid for the specific intent of
initiating the transition from microwave ovens exciting individual
molecules of a liquid such as water, to a new generation of equipment
that disrupts the magnecular bond between molecules. The
development of the latter equipment is evidently prohibited by the
conventional notion of "H-bridges" due to their pure nomenclature
character without quantitative treatment. By comparison magnecular
bonds in a liquid can indeed be treated quantitatively. Additionally,
all magnetic effects are known to have a temperature at which they
disappears (the Curie temperature).
A new equipment that disrupts the magnecular bond between molecules can
be attempted in a number of ways, e.g., via microwaves causing the
magnecular Curie temperature at the microscopic level of individual
molecular couplings, which equipment is currently under development
by the industry. One of the most important possible application of
these advances is a basically new cure for cancer indicated in Figure
4.30.
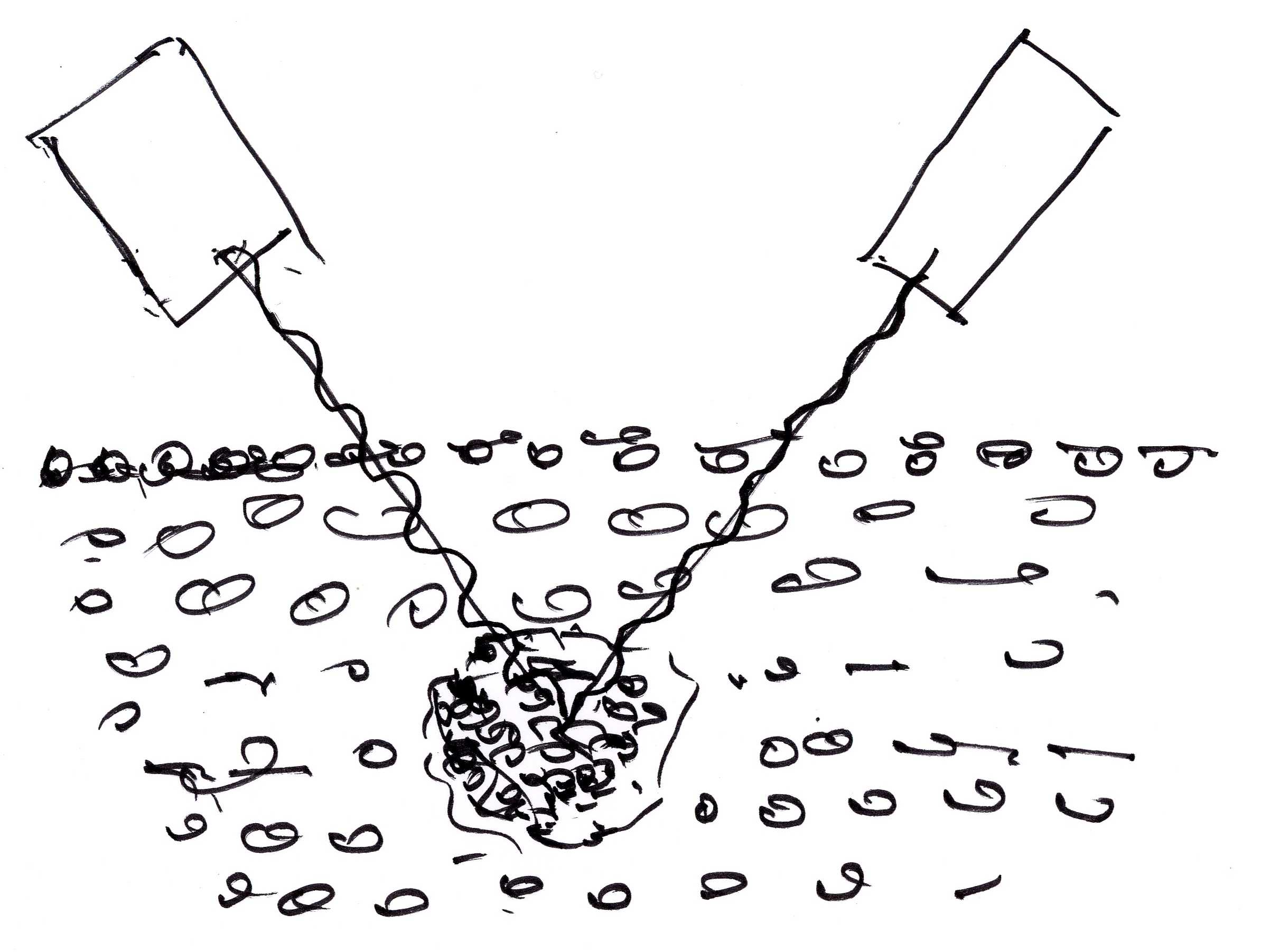
Figure 4.30: Santilli's original hand drawing illustrating a
possible future elimination of cancer. The principle is the disruption
of the magnecular bond between molecules, whether within a cell, a DNA
or other structures depending on the case at hand. In the event the
procedure is possible, it is predicted not to require surgery because
the achievement of the Curie temperature for the disruption of
magnecular bonds in a DNA can be achieved by two microwaves that are
individually non-disruptive for human tissues, and are disruptive
solely at their intersection, as illustrated in the figure. We regret
the inability to report the ongoing industrial research in the field to
prevent academic disruptions.
4.5H. Cloonan's advances in Santilli Magnecules
The cplex-isoelectronic theory is a new theory of pericyclic chemical
reactions and aromatic molecules, within the field of organic chemistry,
which is based on an expansion of the Robinson electronic theory of
organic chemistry. The cplex-isoelectronic theory is a qualitative and
regularity based theory (see
http://www.cplex-isoelectronic.com)
The theory was developed as a response to the complexity of chemistry
due to the inability of the Schrdinger equation to be solved precisely
for H2 and larger molecules, the resultant use of assumptions and
approximations, the formidable and intractable calculations due to the
diversity of factors involved and the effect of chaos on complex
systems.
The new theory makes different predictions from the present quantum
chemical methods and the experimental data, when available, is found to
be consistent with these new predictions. See
Application of the Cplex-isoelectronic theory to electrocyclisations,
sigmatropic rearrangements, cheletropic reactions and antiaromaticity:
Consistent with Santilli's hadronic chemistry.
M. O. Cloonan Int J Hydrogen Energy, Vol. 32, pages 3026-3039 (2007)
These new predictions include the existence of suprafacial concerted
thermal [2 + 2], [4 + 4], [6 + 2] and [6 + 6] cycloadditions,
suprafacial concerted photochemical [4+2] and [6+4] cycloadditions,
stepwise [2+2+2] cycloadditions of ethyne, diamagnetic ring currents for
some cyclic systems with 4np electrons, a stepwise pathway for the
conrotatory photochemical ring opening of 1,3-cyclohexadiene, a
concerted photochemical electrocyclisation for 1,3-cyclohexadiene via
disrotatory motion, a concerted suprafacial [1,5] sigmatropic shift with
inversion for norcaradiene, a concerted suprafacial [1,3] carbon shift
with inversion and retention, a concerted suprafacial photochemical
[1,5] hydrogen migration, a concerted photochemical [3,3] shift,
stabilisation of cyclic 4np electron systems by delocalisation and their
excess energy is due only to electronic repulsion and strain, the
monohomocyclopropenium and cyclopropenyl cations are not ëaromaticí (see
the above quoted reference).
These findings are also consistent with Santilliís Hadronic
Chemistry which predicts more serious limitations with quantum chemistry
especially as the explanation provided by the cplex-isoelectronic theory
is completely different from the rational provided by quantum theories
for pericyclic reactions and aromatic molecules. Nobel prizes have been
awarded for these quantum chemical methods; namely the Woodward Hoffmann
Conservation of Orbital Symmetry, Fukui's Frontier Molecular Orbital
Theory in 1981 and the ab initio and DFT methods in 1998. Thus the way
is paved for new ideas and theories in organic chemistry and thus in
biology. Furthermore it highlights the complexity of chemistry and
biology, the limitations of quantitative theories and the fact that
quantitative science will never admit final descriptions.
Our research into the magnecular bond has confirmed
Santilliís claims that magnecules cannot be detected by infrared (IR)
and nuclear magnetic resonance (NMR) spectroscopy as well as
highlighting some of the reported anomalies from a chemistís vista
[reference 4]. Research is ongoing to explore the magnecular bond by
separation of the magnecules and their structural elucidation by gas
electron diffraction. See
A new electronic theory of pericyclic chemistry and aromaticity is
proposed: The Cplex-isoelectronic theory. Consistent
with Santilli's hadronic chemistry.
M. O. Cloonan, Int J Hydrogen Energy, Vol. 32, pages 159-171 (2007)
**********************************
|
|